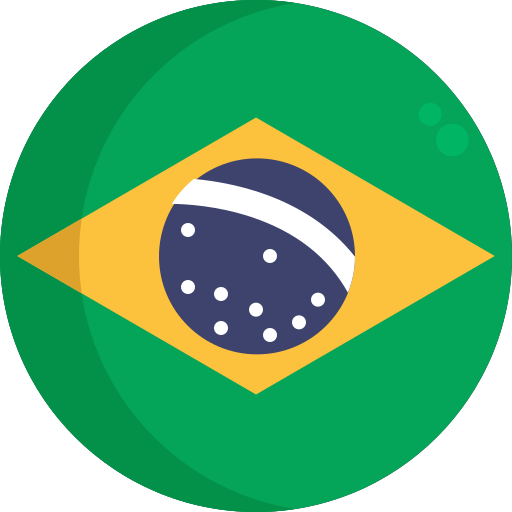
Genetic Structure of the Population of Alternaria solani in Brazil
2010; Wiley; Volume: 159; Issue: 4 Linguagem: Inglês
10.1111/j.1439-0434.2010.01754.x
ISSN1439-0434
AutoresValdir Lourenço, Tatiana Tozzi Martins Souza Rodrigues, Antonio M. D. Campos, Carlos Augusto Dórea Bragança, Klaus Konrad Scheuermann, Ailton Reis, Sérgio Hermínio Brommonschenkel, Luiz A. Maffia, Eduardo S. G. Mizubuti,
Tópico(s)Plant Pathogens and Resistance
ResumoJournal of PhytopathologyVolume 159, Issue 4 p. 233-240 Full Access Genetic Structure of the Population of Alternaria solani in Brazil Valdir Lourenço Jr, Valdir Lourenço Jr Authors' addresses: Depto. de Fitopatologia, Universidade Federal de Viçosa, MG, Brazil Present address: Instituto Agronômico do Paraná (IAPAR). Rod. Celso Garcia Cid Km 375, CEP: 86047-902, Londrina, PR, BrazilSearch for more papers by this author Tatiana T. M. S. Rodrigues, Tatiana T. M. S. Rodrigues Authors' addresses: Depto. de Fitopatologia, Universidade Federal de Viçosa, MG, BrazilSearch for more papers by this author Antonio M. D. Campos, Antonio M. D. Campos Authors' addresses: Depto. de Fitopatologia, Universidade Federal de Viçosa, MG, BrazilSearch for more papers by this author Carlos A. D. Bragança, Carlos A. D. Bragança Authors' addresses: Depto. de Fitopatologia, Universidade Federal de Viçosa, MG, BrazilSearch for more papers by this author Klaus K. Scheuermann, Klaus K. Scheuermann Authors' addresses: Depto. de Fitopatologia, Universidade Federal de Viçosa, MG, Brazil Present address: Epagri, Itajaí, SC, BrazilSearch for more papers by this authorAilton Reis, Ailton Reis Present address: Instituto Agronômico do Paraná (IAPAR). Rod. Celso Garcia Cid Km 375, CEP: 86047-902, Londrina, PR, BrazilSearch for more papers by this author Sérgio H. Brommonschenkel, Sérgio H. Brommonschenkel Authors' addresses: Depto. de Fitopatologia, Universidade Federal de Viçosa, MG, BrazilSearch for more papers by this author Luiz A. Maffia, Luiz A. Maffia Authors' addresses: Depto. de Fitopatologia, Universidade Federal de Viçosa, MG, BrazilSearch for more papers by this author Eduardo S. G. Mizubuti, Eduardo S. G. Mizubuti Authors' addresses: Depto. de Fitopatologia, Universidade Federal de Viçosa, MG, BrazilSearch for more papers by this author Valdir Lourenço Jr, Valdir Lourenço Jr Authors' addresses: Depto. de Fitopatologia, Universidade Federal de Viçosa, MG, Brazil Present address: Instituto Agronômico do Paraná (IAPAR). Rod. Celso Garcia Cid Km 375, CEP: 86047-902, Londrina, PR, BrazilSearch for more papers by this author Tatiana T. M. S. Rodrigues, Tatiana T. M. S. Rodrigues Authors' addresses: Depto. de Fitopatologia, Universidade Federal de Viçosa, MG, BrazilSearch for more papers by this author Antonio M. D. Campos, Antonio M. D. Campos Authors' addresses: Depto. de Fitopatologia, Universidade Federal de Viçosa, MG, BrazilSearch for more papers by this author Carlos A. D. Bragança, Carlos A. D. Bragança Authors' addresses: Depto. de Fitopatologia, Universidade Federal de Viçosa, MG, BrazilSearch for more papers by this author Klaus K. Scheuermann, Klaus K. Scheuermann Authors' addresses: Depto. de Fitopatologia, Universidade Federal de Viçosa, MG, Brazil Present address: Epagri, Itajaí, SC, BrazilSearch for more papers by this authorAilton Reis, Ailton Reis Present address: Instituto Agronômico do Paraná (IAPAR). Rod. Celso Garcia Cid Km 375, CEP: 86047-902, Londrina, PR, BrazilSearch for more papers by this author Sérgio H. Brommonschenkel, Sérgio H. Brommonschenkel Authors' addresses: Depto. de Fitopatologia, Universidade Federal de Viçosa, MG, BrazilSearch for more papers by this author Luiz A. Maffia, Luiz A. Maffia Authors' addresses: Depto. de Fitopatologia, Universidade Federal de Viçosa, MG, BrazilSearch for more papers by this author Eduardo S. G. Mizubuti, Eduardo S. G. Mizubuti Authors' addresses: Depto. de Fitopatologia, Universidade Federal de Viçosa, MG, BrazilSearch for more papers by this author First published: 26 October 2010 https://doi.org/10.1111/j.1439-0434.2010.01754.xCitations: 12 Departamento de Fitopatologia, Universidade Federal de Viçosa, Viçosa, MG, Brazil AboutSectionsPDF ToolsRequest permissionExport citationAdd to favoritesTrack citation ShareShare Give accessShare full text accessShare full-text accessPlease review our Terms and Conditions of Use and check box below to share full-text version of article.I have read and accept the Wiley Online Library Terms and Conditions of UseShareable LinkUse the link below to share a full-text version of this article with your friends and colleagues. Learn more.Copy URL Abstract Understanding the genetic structure of the population of Alternaria solani (AS) is an important component of epidemiological studies of early blight, a severe disease that affects potato (Po) and tomato (To) worldwide. Up to 150 isolates obtained from both hosts were analysed with RAPD and AFLP markers to estimate the amount and distribution of genetic variability of AS in Brazil. Using RAPD, gene diversity (h = 0.20) and scaled indices of diversity of Shannon (H′ = 0.66) and Stoddart and Taylor's (G = 0.31) for the Po population were higher than those of the To (h = 0.07, H′ = 0.34, G = 0.17). For AFLP, the statistics for the Po (h = 0.17, H′ = 0.86, G = 0.49) and To (h = 0.17, H′ = 0.85, G = 0.36) populations were similar. For each RAPD and AFLP locus, the allele frequency for the overall population ranged from 0.006 to 0.988, and 0.007 to 0.993, respectively. Genetic differentiation was high (GST = 0.41 and θ = 0.59) and moderately high (GST = 0.23 and θ = 0.37) when estimated with RAPD and AFLP, respectively. Based on cluster analyses, there was strong evidence of association of pathogen haplotypes with host species. The null hypothesis of random association of alleles was rejected in the analysis of both RAPD (IA = 13.1, P < 0.001) and AFLP (IA = 2.2, P < 0.001) markers. The average number of migrants was estimated to be around one and two individuals per generation, using RAPD and AFLP, respectively. There was no correlation between genetic distance and geographical origin of AS haplotypes for RAPD (r = −0.07, P = 0.84) and AFLP (r = −0.03, P = 0.70). The AS population is clonal with high genetic variability, and there is genetic differentiation between the populations that affect To and Po. Introduction The amount and distribution of genetic variability of plant pathogen populations affect the efficacy of control strategies of plant diseases (McDonald and Linde 2002). The efficacy of both resistant plant varieties and fungicides can be directly impacted by the genetic variability of pathogens (Milgroom and Peever 2003). Thus, the investigation of the genetic structure of pathogen populations should be an important step when planning research programs aimed at plant disease management. Alternaria solani Sorauer is a mitosporic fungus associated with early blight, a plant disease that occurs worldwide wherever potatoes and tomatoes are grown (Pscheidt and Stevenson 1986; Rotem 1994). Early blight epidemics are particularly severe in tropical countries during warm and wet seasons (Batista et al. 2006; Mantecón 2007). Nevertheless, the disease is becoming more severe in all regions partly due to warmer temperatures experienced worldwide (Kapsa 2008). Although wild Solanum species are natural reservoirs of resistance genes to early blight (Jansky and Rouse 2003; Chaerani et al. 2007), large-scale planting of early blight-resistant tomato or potato cultivars with good agronomic/commercial characteristics is not common. In the tropics, the control of early blight relies mostly on the intensive use of fungicides (Batista et al. 2006). Thus, the integrated management of the disease on both potato and tomato crops is important but the limited information regarding the genetic variability of A. solani hinders the efficacy of control measures. High genetic variability of populations of A. solani is reported in several countries (Petrunak and Christ 1992; Martínez et al. 2004; van der Waals et al. 2004; Kumar et al. 2008). In the USA, high genetic diversity between isolates from potato and tomato plants was detected using isozymes (Petrunak and Christ 1992) and RAPD (Weir et al. 1998) as well as significant differences between isolates from the USA and from other countries (Weir et al. 1998). Similarly, high genetic variability among South African isolates from potato was inferred after analysing random amplified microsatellites markers (van der Waals et al. 2004). Genetic differentiation and evidence of host specificity of A. solani isolates from Cuba, Turkey, Russia, USA, Greece and Brazil were detected using the AFLP marker (Martínez et al. 2004). Unfortunately, only eight Brazilian isolates, all sampled from potato and from a single location in Minas Gerais State, prevented broader inferences regarding the population of a pathogen that can affect more than one host and is widely distributed in the country. During the 1990s, few studies were conducted to assess variation of A. solani in Brazil, using classical markers. A reduced set of ten isolates from potato and tomato plants, all from São Paulo State, were compared based on pathogenicity, isozyme analysis, and fungicide sensitivity tests (Fancelli 1991). Differences in aggressiveness when inoculated on tomato plants and physiological and genetic variability were detected in isolates from different host species. In a previous work, genetic differentiation between A. solani associated with potato and tomato plants was detected with RAPD markers of isolates collected from different regions of Brazil (Scheuermann et al. 2004). This motivated an expanded investigation to quantify the amount of genetic variation in the population of A. solani and its distribution in Brazil. To allow for reliable inferences, the genetic structure should be determined based on more than one marker. Despite the low reproducibility of RAPD, this marker is commonly used to gather preliminary information about the genetic variation (Schlotterer 2004; O'Gorman et al. 2008). Combined with AFLP, a more reproducible marker, more regions of the genome can be scanned and a more accurate assessment of genetic variation can be accomplished (Schlotterer 2004). In this study, the genetic diversity within and between populations of A. solani associated with potato and tomato plants, sampled from a wide geographic range in Brazil, were determined using both RAPD and AFLP markers. The objectives of the study were to evaluate (i) gene and genotypic diversity of A. solani; (ii) how genetic variation is spatially distributed and whether genetic variation is related to the geographical origin of isolates; and (iii) the evidence of association of A. solani isolates to potato and tomato crops. Materials and Methods Sampling, isolation and DNA extraction Diseased potato and tomato leaves or fruits (one to 20 samples per field) were collected in several producing regions (Fig. 1). Samples were temporarily stored in an insulated box ('cooler') and taken to the laboratory where isolation procedures were carried out. Pure monoconidial cultures of A. solani were obtained after direct or indirect isolation from diseased tissues (Dhingra and Sinclair 1995). The monoconidial isolates were preserved using four methods: filter paper stored at −20°C (Borromeu et al. 1993), mineral oil, sterilized distilled water and silica gel. The last three were maintained in 10-ml flasks that were stored at room temperature (Dhingra and Sinclair 1995). Isolates obtained from potato or tomato plants are hereafter referred to as 'potato or tomato isolates' and were assumed to belong to a 'potato population' or 'tomato population'. Figure 1Open in figure viewerPowerPoint Sampled areas (dots) located in potato and tomato producing regions in Brazil. From each area, diseased leaves (potato and tomato) or fruits (tomato) were collected. The sampling sites were georeferenced with a portable GPS device. States from which samples were collected are shaded For DNA extraction, the isolates were grown in liquid medium (10 g sucrose, 2 g l-asparagin, 2 g yeast extract, 15 mm KH2PO4, 0.4 mm MgSO4.7H2O, 1.5 μm ZnSO4.7H2O, 1.8 μm FeCl3.6H2O, and 2.5 μm MnCl2. H2O) in Erlenmeyer flasks under continuous agitation at 26°C (Alfenas 1998). After 7 days, the mycelium was washed with distilled water, transferred to filter paper to dry, and macerated in a mortar with liquid nitrogen. The extraction was conducted using a Cetyl trimethylammonium bromide (CTAB)-based protocol (Murray and Thompson 1980). Depending on the size of the DNA pellet, 30–50 μl of TE buffer with RNAse was added and resuspended at 37°C for 2 h. Sample integrity was checked by visual inspection after electrophoresis in agarose gel at 1% with 0.15 μg/ml of ethidium bromide. The quantity of DNA was measured using the lambda phage as molecular weight. RAPD and AFLP analyses For RAPD analysis, PCR was performed in a final volume of 15 μl with 20 ηg of DNA, 1.5 mm of MgCl2, 1 U of Taq DNA polymerase, 0.5 mm of primer, and 0.2 mm of dATP, dCTP, dGTP, and dTTP. Primers P203 (5′CACGGCGAGT 3′), P284 (5′CAGGCGCACA 3′) (Weir et al. 1998), OPC15 (5′GACGGATCAG 3′), OPE14 (5′TGCGGCTGAG 3′), and OPE20 (5′AACGGTGACC 3′) (Scheuermann et al. 2004) were selected for diversity analyses. These RAPD primers were fully reproducible in previous analyses of A. solani isolates. Each amplification reaction consisted of 40 cycles at 94°C for 30 s (denaturation), 35°C for 1 min (annealing), 72°C for 1 min (extension), and a final extension at 72°C for 7 min (Scheuermann et al. 2004). Amplified fragments were separated by agarose gel electrophoresis. For AFLP analyses, the genomic DNA was digested with restriction endonucleases EcoRI and MseI, and adapters of double strand were linked at the ends of the fragments (AFLP Microorganism Primer kit; Invitrogen, Carlsbad, CA, USA). The PCR products from the preamplification reaction were diluted and used as templates to the selective amplification using primers E+AC/M+A, E+AC/M+G, E+AC/M+C, E+AA/M+A, E+AA/M+G, E+AA/M+C, and E+AT/M+A. Amplified fragments were separated by polyacrilamide gel electrophoresis and silver nitrate-stained (Creste et al. 2001). The primer combinations used for genetic structure analyses were selected based on the degree of polymorphism, band strength and reproducibility. The pattern of both RAPD and AFLP polymorphic bands was visually analyzed. Binary matrices were constructed by recording the presence (1) or absence (0) of amplified fragments. As RAPD and AFLP bands of the same size obtained from each primer were considered identical, only reproducible amplified fragments were scored. Both RAPD and AFLP bands were treated as loci, and the presence or absence of amplified fragments as alleles. Data analyses Genetic variability was assessed by quantifying gene and genotypic diversity, genetic distance and genetic differentiation between A. solani populations from potato and tomato. The maximum likelihood ratio test (G2) (Sokal and Rohlf 1981) and gene diversity (h) (Nei 1972) were estimated using POPGENE (Yeh et al. 1997). Multilocus genotypic diversity was estimated with the Shannon–Wiener's H′ and Stoddart and Taylor's G indices (Hill 1973; Stoddart and Taylor 1988). Bootstrapping was conducted using 1000 resamples at a confidence interval of 95%. To compare the diversity indices calculated for populations with different sample sizes, Shannon–Wiener's H′ and Stoddart and Taylor's G indices were scaled by the expected number of genotypes for the smallest sample size being compared (Grünwald et al. 2003). The expected number of genotypes was estimated based on rarefaction curves. The diversity indices and the rarefaction procedure were calculated with the R package (R Development Core Team, 2007). A dendrogram was constructed based on Nei-Li genetic distance matrix using the Unweighted Pair Group Method with Arithmetic Mean as the clustering algorithm (Nei 1987). Statistical support for dendrogram branches were calculated using the WinBoot program (Yap and Nelson 1996) with 1000 bootstrapped samples. The analysis of molecular variance (amova), implemented in the Arlequin 3.11, was used to estimate the subdivision of the population (ΦST) (Excoffier et al. 2005). The coefficients of genetic differentiation (GST) and gene flow estimates (Nm) between A. solani populations were calculated using POPGENE. In addition, genetic differentiation between populations was tested using theta (θ) estimate of Weir and Cockerham, with the generation of 1000 randomized data sets as described in the program Multilocus 1.3. (Agapow and Burt 2001). The relationship between genetic diversity and geographical distance was evaluated by the Mantel's test (Mantel 1967) implemented in Arlequin 3.11. The Mantel test was carried out using 1000 random permutation tests. Finally, to infer about random mating in the population, the index of association (IA) test was performed with Multilocus 1.3. Results Genetic diversity of 168 and 140 isolates was assessed with RAPD and AFLP markers, respectively (Table 1). Each marker was analysed using approximately equal number of isolates from both hosts. Amplification problems of some samples prevented the use of the same number of isolates of both populations. A multilocus data set was constructed combining RAPD matrices using SNAP Combine (Aylor et al. 2006). The combinations of AFLP primers E+AC/M+G, E+AC/M+C, E+AA/M+A, E+AA/M+G, E+AA/M+C were monormophic, and E+AC/M+A was highly variable in a preliminary test (data not shown). The combination E+AT/M+A was considered the most suitable for genetic analyses. With this combination, more than 70 amplified fragments were detected, although only intense and unambiguous bands were scored and used in the study. A total of 39 and 17 haplotypes was generated with the AFLP and RAPD markers, respectively (Table 1). Isolates with the same RAPD and AFLP band pattern were considered to belong to the same haplotype. Table 1. Isolates of Alternaria solani collected from potato and tomato producing regions in Brazil Region/Statea Municipality Date collected Haplotypesb (number of isolates from potato – P or tomato – T) RAPD AFLP SE/MG Araxá 2005 H4 (1P), H7 (1P) H37 (1P), H6 (1P) Bom Repouso 2006 H6 (1P), H7 (3P) H5 (1P), H6 (2P), H37 (2P) Bueno Brandão 2004, 2006 H7 (6P), H9 (1P) H4 (1P), H6 (1P), H37 (2P) Cajuri 2000 H1 (2T) H14 (1T), H29 (1T) Camanducaia 2006 H7 (2P), H9 (1P) H27 (1P), H35 (2P) Carandaí 2005 H1 (7T), H5 (1P), H7 (1P), H11 (1T) H6 (1P), H29 (2T) Coimbra 2000 H1 (2T) H8 (1T) Conselheiro Lafaiete 2005 H1 (3T, 5P), H11 (1T) H12 (1T), H19 (1P), H22 (3P, 2T), H23 (1T), H32 (1T) Ibiá 2005 H7 (4P) H6 (2P), H35 (1P) Igarapé 2006 H1 (1T) N/A Ipuiúna 2006 H7 (6P), H17 (1P) H19 (1P), H37 (1P), H38 (1P), H39 (1P) Ituverava 2005 H1 (1T) H19 (1T) Jaíba 2005 H1 (2T), H12 (1T) H19 (3T) Monsenhor Izidro 2005 H7 (1P), H17 (1P) H33 (1P), H37 (2P) Poços de Caldas 2000 H9 (1P) H6 (1P) Prudente de Morais N/A H1 (1T) H9 (1T) Ressaquinha 2005 H1 (4T) H19 (3T), H29 (1T) Teixeiras N/A H15 (1P) H3 (1P) Tocantins 2000 H1 (1T) H8 (1T) Viçosa 2000 H1 (2T, 1P) H8 (2T), H15 (1P) SE/RJ Bom Jardim 2000 H1 (1T) H24 (1T) Cordeiro 2000 H1 (1T) H17 (1T) Itaperuna 2000 H11 (1T) H13 (1T) Sumidouro 2005 H1 (4T) H29 (1T) Vassouras 2000 H1 (2T), H11 (1T) H8 (1T), H28 (1T) SE/SP Capão Bonito 2000 H2 (1T) H10 (1T) Ibiúna 2005 H1 (3P) H18 (2T) Itapetininga 2005 H1 (5T), H7 (3P) H1 (1P), H19 (3T), H29(1T), H37 (1P), H39 (1P) Marília N/A H13 (1T) H37 (1T) SE/ES Castelo 2005 H1 (1T) N/A Domingos Martins 2005 H1 (3T, 1P) H21 (1P), H22 (1T), H25 (1T) Marechal Floriano 2005 H1 (1T) H19 (2T) Venda Nova do Imigrante 2005 H1 (1T) H19 (1T) S/PR Araucária 2005 H1 (2T) H21 (1P), H30 (1T), H31 (1T) Colombo 2005 H7 (1T) H23 (1T) Contenda 2005 H7 (4P), H8 (1P) H20 (1P), H33 (2P), H35 (1P), H37 (1P) Ponta Grossa 2000 H1 (1T, 1P), H5 (1P), H10 (1T) H3 (2P), H7 (1T) São Mateus do Sul 2000 H7 (1P), H9 (1P) H37 (1P) S/RS Caxias do Sul 2005 H1 (1T) N/A Pelotas 2005 H1 (3T) H11 (1T), H22 (1T), H25 (1T), H26 (1T) São Francisco de Paula 2005 H7 (3P) H35 (1P), H37 (2P) Taquara N/A H1 (1T) H29 (1T) S/SC Alfredo Wagner 2005 H1 (1T), H15 (1T) H28 (1T), H36 (1T) Caçador 2005 H11 (1T) N/A Lages do Régis 2005 H1 (1T) H25 (1T) Rancho Queimado 2006 H1 (2T), H11 (1T) H19 (2T), H29 (1T) CW/DF Brasília 2005 H1 (7P, 1T), H3 (1T), H11 (1T), H13 (1P), H14 (1P) H2 (1T), H16 (2P), H19 (4P), H25 (1T), H37 (1P), H39 (1P) Planaltina 2005 H1 (14T), H16 (1T) H19 (5T), H29 (5T) CW/GO Cristalina 2005 H6 (1P), H7 (4P) H6 (1P), H35 (4P), H37 (1P) Planaltina de Goiás N/A H1 (1T) H29 (1T) CW/TO Colméia 2005 H1 (1T) H19 (1T) NE/BA Mucugê 2005 H6 (1P), H7 (2P) H1 (1P), H34 (1P), H35 (1P) NE/CE Guaraciaba do Norte 2005 H1 (2P), H7 (2P) H1 (2P), H29 (1P), H39 (1P) N/A, not available. aStates: PR (Paraná), RJ (Rio de Janeiro), MG (Minas Gerais), BA (Bahia), SP (São Paulo), ES (Espírito Santo), GO (Goiás), SC (Santa Catarina), RS (Rio Grande do Sul), CE (Ceará), DF (Distrito Federal), and TO (Tocantins); Regions: S (South), SE (Southeast), NE (Northeast), and CW (Central-West). bRAPD and AFLP haplotype codes. The number of isolates and the host of origin, potato (P) or tomato (T) are indicated in parentheses. Isolates with the same RAPD and AFLP banding pattern were considered to belong to the same haplotype. A high percentage of polymorphic loci were estimated with both RAPD and AFLP. With RAPD, the number of scored loci was 55, all polymorphic. With AFLP, 24 loci were scored and 87.5% were polymorphic. High genetic diversity was detected in all populations (Table 2). Gene diversity and scaled genotypic indices values estimated based on RAPD data were higher for the potato than for the tomato population. For AFLP, these statistics had similar values for both populations (Table 2). Table 2. Gene diversity (h) and scaled indices of genotypic diversity measured by Shannon–Wiener (H′) and Stoddart and Taylor's (G) estimated for RAPD and AFLP dataset among Alternaria solani populations from potato and tomato plants Indexes RAPD AFLP Tomato Potato Total Tomato Potato Total h 0.066 (0.0690)a 0.198 (0.2059) 0.215 (0.2060) 0.160 (0.1395) 0.173 (0.1942) 0.217 (0.2040) H′ 0.34 (0.28–0.47)b 0.66 (0.60–0.77) 0.67 (0.60–0.81) 0.85 (0.81–0.92) 0.86 (0.84–0.91) 1.02 (1.01–1.06) G′ 0.17 (0.15–0.19) 0.31 (0.26–0.37) 0.31 (0.27–0.36) 0.36 (0.30–0.47) 0.49 (0.42–0.59) 0.65 (0.55–0.82) aStandard deviation. bNumber in parentheses indicate the lower and upper limits of 95% confidence intervals of the indices estimates. The allele frequencies differed between potato and tomato populations (Table S1). At each RAPD and AFLP locus, the allele frequency for the overall population ranged from 0.006 to 0.988, and from 0.007 to 0.993, respectively. The heterogeneity of allele frequencies across A. solani populations for the RAPD and AFLP was significant in 29 and 11 loci, respectively (Table S1). There was strong evidence of genetic differentiation between tomato and potato populations. Genetic differentiation estimated by the average GST was high (Table S1) and the θ statistics estimated for RAPD and AFLP were 0.59 and 0.37, respectively (P<0.001). For RAPD, the percentage of genetic variation between populations (54.3) was higher than within populations (45.7). The opposite was recorded for the AFLP marker with 37 and 63% of the genetic variation due to variability between and within populations, respectively. There was evidence of association of A. solani haplotypes with potato and tomato plants (2, 3). Two clusters of isolates were formed when analysing the RAPD data (Fig. 2). Cluster 1 was composed mostly by haplotypes associated with potato plants (50 isolates). Only 11 haplotypes in cluster 1 were from tomato plants. Cluster 2 was mostly composed by haplotypes associated with tomato plants (81 isolates) with some haplotypes sampled from potato plants (25 isolates). The haplotype H3 did not group with either cluster 1 or 2 (Fig. 2). Figure 2Open in figure viewerPowerPoint Dendrogram generated by the unweighted pair group method with arithmetic average analysis based on Nei-Li genetic distance matrix from RAPD among Alternaria solani haplotypes (H) from potato and tomato plants. Number in parentheses is the haplotype frequency from potato (P) and tomato (T) plants. Numbers shown above branches represent percentage confidence limits obtained by bootstrap analyses with 1,000 replicates. Bootstrap values below 50% are not shown Figure 3Open in figure viewerPowerPoint Dendrogram generated by the unweighted pair group method with arithmetic average analysis based on Nei-Li genetic distance matrix from AFLP among Alternaria solani haplotypes (H). Number in parentheses is the haplotype frequency from potato (P) and tomato (T) plants. Numbers shown above branches represent percentage confidence limits obtained by bootstrap analyses with 1,000 replicates. Bootstrap values below 50% are not shown Based on AFLP markers, the isolates were grouped into three clusters (Fig. 3). Clusters 1 and 3 had mostly haplotypes from tomato plants with 3 and 63 isolates, respectively. Cluster 2 included mostly the haplotypes associated with potato plants (49 isolates), and only two haplotypes from tomato plants. The haplotype H2 did not group with cluster 1, 2 and 3 (Fig. 3). There was no evidence of recombination in populations of A. solani. The null hypothesis of random association of alleles was rejected for both RAPD (IA =13.1, P < 0.001) and AFLP (IA =2.2, P < 0.001) analyses. Moderate gene flow is occurring between A. solani populations with the average number of migrants estimated around one and two individuals per generation for RAPD and AFLP, respectively (Table S1). There was no correlation between genetic distance and geographical origin of A. solani haplotypes for either RAPD (r = −0.07, P = 0.84) or AFLP (r = −0.03, P = 0.70). Discussion The Brazilian population of A. solani is genetically variable, but there is no evidence of occurrence of random mating, and there are groups of isolates associated with the two economically important host plants. Due to the lack of a known sexual stage in the life cycle of this pathogen (Rotem 1994; Thomma 2003), strong evidence of a clonal population structure and low genotypic diversity were expected. Furthermore, given the almost continuous availability of host plants in several regions in Brazil, one would expect a widespread clone of A. solani throughout the tomato and potato producing zones and low genetic differentiation of the pathogen population according to the host plant. However, two rather unexpected results arose in this study: the genetic diversity of populations of A. solani was relatively high, and there was strong evidence of host association in the pathogen population. Therefore, mutation, gene flow and selection are hypothesized as the most important evolutionary mechanisms shaping the variability of the pathogen population in Brazil. Variation in the population of A. solani is expected to be largely influenced by mutation rather than recombination processes. Although recombination was inferred in populations of some Alternaria species (Berbee et al. 2003), and low values of IA were calculated for Alternaria brassicicola using AFLP markers (Bock et al. 2005), there is no evidence of recombination occurring in the population of A. solani in Brazil. No sexual structures are known to be formed under field conditions, neither has parasexual recombination been reported. Additionally, according to the genetic analyses carried out in the present study, the null hypothesis of a randomly mating population was rejected by the IA test. This fact is corroborated by the mycelial compatibility analyses of A. solani isolates that did not produce sexual structures in any pairing (including some isolates used in the present study). Furthermore, only one mating type was detected among these isolates (Rodrigues et al. 2008). All lines of evidences suggest that mutation plays an important role in generating a diverse population. However, quantification of the mutation rate would be more properly accomplished using DNA sequence data. Another potential source of variation is the presence of transposable elements in A. solani. In other fungal species, these elements result in high mutation rates (Daboussi and Capy 2003). Unfortunately, the contribution of insertion sequences or transposable elements to the variability in A. solani has not been studied and further investigation on this topic is warranted. Gene flow takes place between tomato and potato fields preventing geographic subdivision, but not genetic differentiation of the pathogen population according to the host crops. Aerial conidia are the main type of inoculum for early blight epidemics in both potato and tomato fields, and the close proximity between fields of these two crops in Brazil favor the exchange of A. solani haplotypes (Batista 2006). This can explain the grouping of some A. solani haplotypes from potato and tomato plants in the same RAPD and AFLP clusters. Possibly, a putative interaction between gene flow and selection influenced the structure of A. solani populations in Brazil. Migration allows movement of individuals between hosts, but selection prevents the expected homogenizing effect due to gene flow. Similar results were obtained in another study using the same set of A. solani isolates when gene flow between A. solani populations was inferred from gene ge
Referência(s)