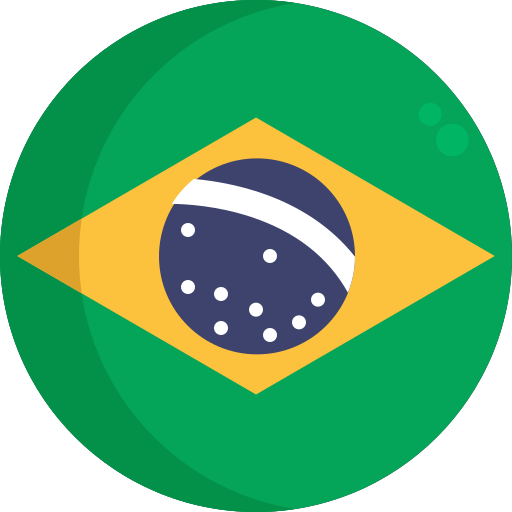
Selective Cathepsin S Inhibition Attenuates Atherosclerosis in Apolipoprotein E–Deficient Mice with Chronic Renal Disease
2015; Elsevier BV; Volume: 185; Issue: 4 Linguagem: Inglês
10.1016/j.ajpath.2014.11.026
ISSN1525-2191
AutoresJose‐Luiz Figueiredo, Masanori Aikawa, Chunyu Zheng, Jacob Aaron, Lilian Lax, Peter Libby, José Luiz de Lima Filho, Sabine Gruener, Jürgen Fingerle, Wolfgang Haap, Guido Hartmann, Elena Aïkawa,
Tópico(s)GDF15 and Related Biomarkers
ResumoChronic renal disease (CRD) accelerates the development of atherosclerosis. The potent protease cathepsin S cleaves elastin and generates bioactive elastin peptides, thus promoting vascular inflammation and calcification. We hypothesized that selective cathepsin S inhibition attenuates atherogenesis in hypercholesterolemic mice with CRD. CRD was induced by 5/6 nephrectomy in high-fat high-cholesterol fed apolipoprotein E–deficient mice. CRD mice received a diet admixed with 6.6 or 60 mg/kg of the potent and selective cathepsin S inhibitor RO5444101 or a control diet. CRD mice had significantly higher plasma levels of osteopontin, osteocalcin, and osteoprotegerin (204%, 148%, and 55%, respectively; P < 0.05), which were inhibited by RO5444101 (60%, 40%, and 36%, respectively; P < 0.05). Near-infrared fluorescence molecular imaging revealed a significant reduction in cathepsin activity in treated mice. RO5444101 decreased osteogenic activity. Histologic assessment in atherosclerotic plaque demonstrated that RO5444101 reduced immunoreactive cathepsin S (P < 0.05), elastin degradation (P = 0.01), plaque size (P = 0.01), macrophage accumulation (P < 0.01), growth differentiation factor-15 (P = 0.0001), and calcification (alkaline phosphatase activity, P < 0.01; osteocalcin, P < 0.05). Furthermore, cathepsin S inhibitor or siRNA significantly decreased expression of growth differentiation factor-15 and monocyte chemotactic protein-1 in a murine macrophage cell line and human primary macrophages. Systemic inhibition of cathepsin S attenuates the progression of atherosclerotic lesions in 5/6 nephrectomized mice, serving as a potential treatment for atherosclerosis in patients with CRD. Chronic renal disease (CRD) accelerates the development of atherosclerosis. The potent protease cathepsin S cleaves elastin and generates bioactive elastin peptides, thus promoting vascular inflammation and calcification. We hypothesized that selective cathepsin S inhibition attenuates atherogenesis in hypercholesterolemic mice with CRD. CRD was induced by 5/6 nephrectomy in high-fat high-cholesterol fed apolipoprotein E–deficient mice. CRD mice received a diet admixed with 6.6 or 60 mg/kg of the potent and selective cathepsin S inhibitor RO5444101 or a control diet. CRD mice had significantly higher plasma levels of osteopontin, osteocalcin, and osteoprotegerin (204%, 148%, and 55%, respectively; P < 0.05), which were inhibited by RO5444101 (60%, 40%, and 36%, respectively; P < 0.05). Near-infrared fluorescence molecular imaging revealed a significant reduction in cathepsin activity in treated mice. RO5444101 decreased osteogenic activity. Histologic assessment in atherosclerotic plaque demonstrated that RO5444101 reduced immunoreactive cathepsin S (P < 0.05), elastin degradation (P = 0.01), plaque size (P = 0.01), macrophage accumulation (P < 0.01), growth differentiation factor-15 (P = 0.0001), and calcification (alkaline phosphatase activity, P < 0.01; osteocalcin, P < 0.05). Furthermore, cathepsin S inhibitor or siRNA significantly decreased expression of growth differentiation factor-15 and monocyte chemotactic protein-1 in a murine macrophage cell line and human primary macrophages. Systemic inhibition of cathepsin S attenuates the progression of atherosclerotic lesions in 5/6 nephrectomized mice, serving as a potential treatment for atherosclerosis in patients with CRD. Half of all patients with chronic renal disease (CRD) die of cardiovascular causes.1Campean V. Neureiter D. Varga I. Runk F. Reiman A. Garlichs C. Achenbach S. Nonnast-Daniel B. Amann K. Atherosclerosis and vascular calcification in chronic renal failure.Kidney Blood Press Res. 2005; 28: 280-289Crossref PubMed Scopus (62) Google Scholar, 2Schiffrin E.L. Lipman M.L. Mann J.F. Chronic kidney disease: effects on the cardiovascular system.Circulation. 2007; 116: 85-97Crossref PubMed Scopus (1209) Google Scholar Patients with early stages of CRD who are not undergoing dialysis have cardiovascular disease (CVD) risk similar to that of patients with established coronary artery disease,3Foley R.N. Parfrey P.S. Sarnak M.J. Clinical epidemiology of cardiovascular disease in chronic renal disease.Am J Kidney Dis. 1998; 32: S112-S119Abstract Full Text PDF PubMed Scopus (2991) Google Scholar whereas patients with end-stage renal disease, treated by dialysis, have an approximately 30 times greater CVD risk than the general population.4de Jager D.J. Grootendorst D.C. Jager K.J. van Dijk P.C. Tomas L.M. Ansell D. Collart F. Finne P. Heaf J.G. De Meester J. Wetzels J.F. Rosendaal F.R. Dekker F.W. Cardiovascular and noncardiovascular mortality among patients starting dialysis.JAMA. 2009; 302: 1782-1789Crossref PubMed Scopus (544) Google Scholar, 5Sarnak M.J. Levey A.S. Schoolwerth A.C. Coresh J. Culleton B. Hamm L.L. McCullough P.A. Kasiske B.L. Kelepouris E. Klag M.J. Parfrey P. Pfeffer M. Raij L. Spinosa D.J. Wilson P.W. Kidney disease as a risk factor for development of cardiovascular disease: a statement from the American Heart Association councils on kidney in cardiovascular disease, high blood pressure research, clinical cardiology, and epidemiology and prevention.Hypertension. 2003; 42: 1050-1065Crossref PubMed Scopus (957) Google Scholar Despite being at elevated risk for CVD, patients with CRD have experienced limited benefits from statin treatment alone.6Fellstrom B.C. Jardine A.G. Schmieder R.E. Holdaas H. Bannister K. Beutler J. Chae D.W. Chevaile A. Cobbe S.M. Gronhagen-Riska C. De Lima J.J. Lins R. Mayer G. McMahon A.W. Parving H.H. Remuzzi G. Samuelsson O. Sonkodi S. Sci D. Suleymanlar G. Tsakiris D. Tesar V. Todorov V. Wiecek A. Wuthrich R.P. Gottlow M. Johnsson E. Zannad F. Rosuvastatin and cardiovascular events in patients undergoing hemodialysis.N Engl J Med. 2009; 360: 1395-1407Crossref PubMed Scopus (1622) Google Scholar, 7Baigent C. Landray M.J. Reith C. Emberson J. Wheeler D.C. Tomson C. et al.The effects of lowering LDL cholesterol with simvastatin plus ezetimibe in patients with chronic kidney disease (study of heart and renal protection): a randomised placebo-controlled trial.Lancet. 2011; 377: 2181-2192Abstract Full Text Full Text PDF PubMed Scopus (1921) Google Scholar Hence, the need is emerging to investigate the mechanisms responsible for CVD in CRD patients to develop effective new therapies. Arterial inflammation is a key facet of atherosclerotic lesion initiation and progression.8Hansson G.K. Inflammation, atherosclerosis, and coronary artery disease.N Engl J Med. 2005; 352: 1685-1695Crossref PubMed Scopus (7069) Google Scholar, 9Libby P. Aikawa M. Stabilization of atherosclerotic plaques: new mechanisms and clinical targets.Nat Med. 2002; 8: 1257-1262Crossref PubMed Scopus (499) Google Scholar Several molecular mechanisms participate in the response to injuries at the vascular wall and in the formation and progression of atherosclerotic lesions.10Businaro R. Tagliani A. Buttari B. Profumo E. Ippoliti F. Di Cristofano C. Capoano R. Salvati B. Rigano R. Cellular and molecular players in the atherosclerotic plaque progression.Ann N Y Acad Sci. 2012; 1262: 134-141Crossref PubMed Scopus (46) Google Scholar Chronic inflammation likely accelerates atherosclerosis in patients with CRD,11McIntyre C.W. Harrison L.E. Eldehni M.T. Jefferies H.J. Szeto C.C. John S.G. Sigrist M.K. Burton J.O. Hothi D. Korsheed S. Owen P.J. Lai K.B. Li P.K. Circulating endotoxemia: a novel factor in systemic inflammation and cardiovascular disease in chronic kidney disease.Clin J Am Soc Nephrol. 2011; 6: 133-141Crossref PubMed Scopus (350) Google Scholar and the combination of chronic inflammation and an imbalance in the calcium phosphate serum level in these patients exacerbates these processes.12Navarro-Gonzalez J.F. Mora-Fernandez C. Muros M. Herrera H. Garcia J. Mineral metabolism and inflammation in chronic kidney disease patients: a cross-sectional study.Clin J Am Soc Nephrol. 2009; 4: 1646-1654Crossref PubMed Scopus (75) Google Scholar, 13Chen H.Y. Chiu Y.L. Hsu S.P. Pai M.F. Lai C.F. Yang J.Y. Peng Y.S. Tsai T.J. Wu K.D. Elevated C-reactive protein level in hemodialysis patients with moderate/severe uremic pruritus: a potential mediator of high overall mortality.QJM. 2010; 103: 837-846Crossref PubMed Scopus (50) Google Scholar In addition, CRD patients receiving hemodialysis have elevated levels of circulating proinflammatory cytokines,14Lam M.F. Leung J.C. Lam C.W. Tse K.C. Lo W.K. Lui S.L. Chan T.M. Tam S. Lai K.N. Procalcitonin fails to differentiate inflammatory status or predict long-term outcomes in peritoneal dialysis-associated peritonitis.Perit Dial Int. 2008; 28: 377-384Crossref PubMed Scopus (20) Google Scholar which can initiate and perpetuate the inflammation-calcification loop. Cathepsin S plays a critical role in vascular inflammation and calcification. Monocyte-derived macrophages that mediate vascular inflammation express and secrete the cysteine protease cathepsin S.15Pan L. Li Y. Jia L. Qin Y. Qi G. Cheng J. Qi Y. Li H. Du J. Cathepsin S deficiency results in abnormal accumulation of autophagosomes in macrophages and enhances Ang II-induced cardiac inflammation.PLoS One. 2012; 7: e35315Crossref PubMed Scopus (76) Google Scholar At the basal membrane of the blood vessels, secreted cathepsin S cleaves several extracellular matrix proteins, including laminin, collagen, and, preferentially, elastin, which generate bioactive elastin peptides.16Lohoefer F. Reeps C. Lipp C. Rudelius M. Zimmermann A. Ockert S. Eckstein H.H. Pelisek J. Histopathological analysis of cellular localization of cathepsins in abdominal aortic aneurysm wall.Int J Exp Pathol. 2012; 93: 252-258Crossref PubMed Scopus (28) Google Scholar, 17Samouillan V. Dandurand J. Nasarre L. Badimon L. Lacabanne C. Llorente-Cortes V. Lipid loading of human vascular smooth muscle cells induces changes in tropoelastin protein levels and physical structure.Biophys J. 2012; 103: 532-540Abstract Full Text Full Text PDF PubMed Scopus (15) Google Scholar Elastin-derived peptides and fragments, also known as matrikines, can incite inflammation. Elastin peptides stimulate macrophage chemotaxis18Simpson C.L. Lindley S. Eisenberg C. Basalyga D.M. Starcher B.C. Simionescu D.T. Vyavahare N.R. Toward cell therapy for vascular calcification: osteoclast-mediated demineralization of calcified elastin.Cardiovasc Pathol. 2007; 16: 29-37Crossref PubMed Scopus (41) Google Scholar and promote vascular inflammation and calcification.19Liu J. Sukhova G.K. Sun J.S. Xu W.H. Libby P. Shi G.P. Lysosomal cysteine proteases in atherosclerosis.Arterioscler Thromb Vasc Biol. 2004; 24: 1359-1366Crossref PubMed Scopus (332) Google Scholar In addition, cathepsin S co-localizes with regions of increased elastin breaks in atherosclerotic plaques.20Samokhin A.O. Lythgo P.A. Gauthier J.Y. Percival M.D. Bromme D. Pharmacological inhibition of cathepsin S decreases atherosclerotic lesions in apoe-/- mice.J Cardiovasc Pharmacol. 2010; 56: 98-105Crossref PubMed Scopus (49) Google Scholar We previously reported that cathepsin S deficiency leads to reduced elastolytic activity and decreased vascular inflammation and calcification in the arteries of hypercholesterolemic mice with experimental CRD, providing in vivo evidence implicating cathepsin S–induced elastolysis in arterial and aortic valve calcification.21Aikawa E. Aikawa M. Libby P. Figueiredo J.L. Rusanescu G. Iwamoto Y. Fukuda D. Kohler R.H. Shi G.P. Jaffer F.A. Weissleder R. Arterial and aortic valve calcification abolished by elastolytic cathepsin S deficiency in chronic renal disease.Circulation. 2009; 119: 1785-1794Crossref PubMed Scopus (227) Google Scholar To seek a clinically translatable proof of concept, the present study tested the hypothesis that treatment with a highly selective cathepsin S inhibitor attenuates inflammation and atherosclerotic lesion formation in the arteries of hypercholesterolemic mice with CRD. Male 10-week-old Apoe−/− mice (N = 60) from The Jackson Laboratory (Bar Harbor, ME) were fed a high-fat high-cholesterol diet (Teklad TD.88137; Harlan Laboratories, Indianapolis, IN) for 10 weeks. At 20 weeks of age, mice were randomized either to continue with the diet (n = 15) or to undergo 5/6 nephrectomy (n = 45). CRD mice were then treated with 6.6 or 60 mg/kg of RO5444101, a highly potent and selective cathepsin S inhibitor (Hoffmann-La Roche, Basel, Switzerland) (n = 15 per group), admixed with the high-fat high-cholesterol diet for an additional 10 weeks. The Harvard Medical School Standing Committee on Animals approved all the animal studies. Human peripheral blood mononuclear cells (PBMCs) were isolated by centrifugation in Ficoll-Hypaque (Sigma-Aldrich, St. Louis, MO) and adherence. Cells were cultured for 10 days in RPMI 1640 medium (Invitrogen, Carlsbad, CA) supplemented with 5% heat-inactivated human serum, 2 mmol/L l-glutamine, 100 μg/mL penicillin, and 100 U/mL streptomycin to differentiate into macrophages. Murine macrophage-like RAW264.7 cells were purchased from ATCC (Manassas, VA) and grown in Dulbecco's modified Eagle's medium with 10% fetal bovine serum. We used an established model to induce chronic renal failure by controlling the amount of kidney mass removed.21Aikawa E. Aikawa M. Libby P. Figueiredo J.L. Rusanescu G. Iwamoto Y. Fukuda D. Kohler R.H. Shi G.P. Jaffer F.A. Weissleder R. Arterial and aortic valve calcification abolished by elastolytic cathepsin S deficiency in chronic renal disease.Circulation. 2009; 119: 1785-1794Crossref PubMed Scopus (227) Google Scholar This procedure includes two steps to create uremia.21Aikawa E. Aikawa M. Libby P. Figueiredo J.L. Rusanescu G. Iwamoto Y. Fukuda D. Kohler R.H. Shi G.P. Jaffer F.A. Weissleder R. Arterial and aortic valve calcification abolished by elastolytic cathepsin S deficiency in chronic renal disease.Circulation. 2009; 119: 1785-1794Crossref PubMed Scopus (227) Google Scholar, 22Bro S. Bentzon J.F. Falk E. Andersen C.B. Olgaard K. Nielsen L.B. Chronic renal failure accelerates atherogenesis in apolipoprotein E-deficient mice.J Am Soc Nephrol. 2003; 14: 2466-2474Crossref PubMed Scopus (132) Google Scholar First, we performed 2/3 nephrectomy, removing the top one-third and bottom one-third of the left kidney. Then, after 7 days of healing, the right kidney was removed. Twenty-four hours before imaging, mice received simultaneous i.v. injections of two spectrally distinct molecular imaging agents: a protease-activatable, pan-cathepsin fluorescent agent (ProSense 750; PerkinElmer, Waltham, MA) and a bisphosphonate-conjugated calcium tracer (OsteoSense 680; PerkinElmer). Dual-channel (633 nm for excitation and 748 nm for emission) in vivo near-infrared fluorescence (NIRF) of carotid arteries was acquired using a laser scanning multicolor fluorescence microscope (Olympus Corp, Tokyo, Japan), as previously described elsewhere.23Aikawa E. Nahrendorf M. Figueiredo J.L. Swirski F.K. Shtatland T. Kohler R.H. Jaffer F.A. Aikawa M. Weissleder R. Osteogenesis associates with inflammation in early-stage atherosclerosis evaluated by molecular imaging in vivo.Circulation. 2007; 116: 2841-2850Crossref PubMed Scopus (517) Google Scholar, 24Aikawa E. Nahrendorf M. Sosnovik D. Lok V.M. Jaffer F.A. Aikawa M. Weissleder R. Multimodality molecular imaging identifies proteolytic and osteogenic activities in early aortic valve disease.Circulation. 2007; 115: 377-386Crossref PubMed Scopus (315) Google Scholar For ex vivo NIRF reflectance imaging, we perfused the heart with saline solution to flush out blood. Aortas and arteries were dissected and then were imaged using an NIRF reflectance imaging system (Image Station 4000MM; Eastman Kodak Co., New Haven, CT). Image stacks were processed and analyzed using ImageJ software version 1.41 (NIH, Bethesda, MD). Mice were then sacrificed for correlative histologic analyses of the aorta and arteries. Male 8-week-old wild-type mice (N = 8; Charles River Laboratories, Sulzfeld, Germany) received RO5444101, and terminal blood samples were collected at seven different time points in precooled EDTA-coated tubes. The samples were kept on ice and immediately centrifuged at 4°C to obtain plasma. Quantification of compound levels in plasma was performed by liquid chromatography–tandem mass spectrometry analysis. Increased p10 was confirmed in spleens, which were homogenized in radioimmunoprecipitation assay buffer with protease inhibitors. Lysates were electrophoresed, and proteins were transferred to polyvinylidene difluoride membrane. Membrane was incubated with CD74 primary antibody (BD Pharmingen, Heidelberg, Germany) and then with anti-rabbit secondary antibody. The membrane was developed by Western blot analysis (GE Healthcare, Buckinghamshire, UK). Blood was collected via the inferior vena cava and was spun in a refrigerated centrifuge; serum was stored at −80°C. Serum levels of osteogenic markers, including bone gamma-carboxylglutamate (gla) protein or osteocalcin, secreted phosphoprotein 1 or osteopontin, and osteoprotegerin or tumor necrosis factor receptor superfamily, member 11b, were measured by sandwich enzyme-linked immunosorbent assay kits (Millipore, Billerica, MA). Tissue samples were frozen in optimum cutting temperature compound (Sakura Finetek, Torrence, CA), and 6-μm serial sections were cut and stained with hematoxylin and eosin for general morphologic evaluation. Alkaline phosphatase activity was detected on cryosections (alkaline phosphatase substrate kit; Vector Laboratories, Burlingame, CA). Van Gieson stain was used to assess elastin. Immunohistochemical analysis for macrophages (anti-mouse mac3; BD Biosciences, San Jose, CA), cathepsin S (anti-mouse cathepsin S; Santa Cruz Biotechnology, Santa Cruz, CA), osteocalcin (goat anti-mouse polyclonal antibody; Serotech, Dusseldorf, Germany), and growth differentiation factor-15 (GDF15) (rabbit anti–Gdf-15 polyclonal antibody; Bioss Antibodies, Woburn, MA) was performed using the avidin-biotin peroxidase method. Images were captured and processed using an Eclipse 80i microscope (Nikon Instruments, Melville, NY). Serial or adjacent sections were used for analyses of quantitative data. Double immunofluorescence staining was performed using cathepsin S antibodies and either mac3 or α-smooth muscle actin (clone 1A4; Sigma-Aldrich). Sections were counterstained with DAPI to visualize nuclei. Images were processed using an Eclipse confocal microscope system (Nikon Instruments). RAW264.7 cells were transfected with 200 nmol/L siRNA against cathepsin S or control scrambled nontargeting siRNA (Dharmacon, Lafayette, CO) using Lipofectamine 2000 (Invitrogen) for 48 hours before experiments, following the manufacturer's protocols. By this method, the silencing efficiency was consistently >90%. Total RNA from human and murine macrophages was isolated using an RNeasy kit (Qiagen GmbH, Hilden, Germany) and was reverse transcribed by SuperScript II Reverse Transcriptase (Invitrogen) and oligo (dT) primers. Quantitative PCR was performed in the MyiQ single-color real-time PCR detection system (Bio-Rad Laboratories, Hercules, CA). The following primers from Integrated DNA Technologies (Coralville, IA) were used: hGDF-15: forward 5′-GACCCTCAGAGTTGCACTCC-3′ and reverse 5′-GCCTGGTTAGCAGGTCCTC-3′; mGDF-15: forward 5′-AGCTGCTACTCCGCGTCAA-3′ and reverse 5′-GTAAGCGCAGTTCCAGCTG-3′; hMCP-1/CCL2: forward 5′-CAGCCAGATGCAATCAATGCC-3′ and reverse 5′-TGGAATCCTGAACCCACTTCT-3′; and mMCP-1/CCL2: forward 5′-AGGTCCCTGTCATGCTTCTG-3′ and reverse 5′-TCTGGACCCATTCCTTCTTG-3′. The mRNA levels of the various genes tested were normalized to glyceraldehyde-3-phosphate dehydrogenase levels for human samples and to β-actin levels for murine samples. Enzymatic activity was measured by observing the increase in fluorescence intensity caused by cleavage of a peptide substrate containing a fluorophore, the emission of which is quenched in the intact peptide. The assay buffer consisted of 100 mmol/L potassium phosphate, pH 6.5, 5 mmol/L EDTA-Na, 0.001% Triton X-100 (Roche Diagnostics GmbH, Mannheim, Germany), and 5 mmol/L dithiothreitol. The enzymes (all at 1 nmol/L) used were as follows: human and mouse cathepsins S, K, L, and B were measured. Substrate (20 μmol/L): Z-Val-Val-Arg-AMC, except for cathepsin K, which uses Z-Leu-Arg-AMC (both from Bachem, Bubendorf, Sweden). Excitation was 360 nm, and emission was 465 nm. Enzyme was added to the substance dilutions in 96-well microtiter plates, and the reaction was started with substrate. Fluorescence emission was measured over 20 minutes. Invariant chain p10 accumulation was detected in human B cells by Western blot analysis. B cells were purified from human PBMCs, with further purification of B cells using a CD19+ affinity bead purification kit (Miltenyi Biotec GmbH, Bergisch Gladbach, Germany). Cells were stimulated with indicated concentrations of RO5444101 for 16 hours and then were homogenized in radioimmunoprecipitation assay buffer with protease inhibitors. Lysates were electrophoresed, and proteins were transferred to polyvinylidene difluoride membrane. Membrane was incubated with CD74 primary antibody Pin.1 and goat anti-mouse IgG–horseradish peroxidase (Art. 32430; Pierce Biotechnology, Rockford, IL). The membrane was developed by Western blot analysis (GE Healthcare). Data are presented as means ± SEM. Analysis of variance and Student's t-test were performed using GraphPad Prism software version 5.0 (GraphPad Software Inc., San Diego, CA). This study used RO5444101, an inhibitor with high specificity to cathepsin S but not other cathepsins (Figure 1A). This compound inhibits the active site of cathepsin S with high potency (inhibitory constant was 0.13 nmol/L using an in vitro peptide cleavage assay) and good selectivity over other cathepsins (B, K, L, C, H, V, and X) or noncysteine proteases, tested to concentrations of up to 10 μmol/L (Table 1). The cellular activity of the compound was tested in assays of antigen presentation in human B cells. Cathepsin S cleaves critically the invariant chain, a chaperone of the major histocompatibility complex class II molecule. Inhibition of cathepsin S in antigen-presenting cells results in the accumulation of an intermediate of the invariant chain—p10—as determined by Western blot analysis (Figure 1B). The mean EC50 of RO5444101 was determined to be 189.3 nmol/L (Table 2). We also tested the effects of this compound in mice and observed that it significantly induced p10 accumulation in spleen extracts, demonstrating its in vivo efficacy (Figure 1, C and D). Reduced invariant cleavage, in turn, reduces surface major histocompatibility complex class II levels and the antigen-induced cytokine response in PBMCs. Inhibition of cathepsin S is, therefore, of great interest in chronic inflammatory diseases because it may reduce tissue damage and dampen the generation of autoimmunity. Overall, RO5444101 compound shows good bioavailability and pharmacokinetic properties in mice and cynomolgus monkeys, making it an attractive small molecule inhibitor to study the function of cathepsin S in the context of chronic inflammatory diseases, including atherosclerosis.Table 1Species and Protease Selectivity of the Cathepsin S Inhibitor RO5444101RO compound50% Inhibitory concentration (nmol/L)Human CatSMouse CatSDog CatSRabbit CatSHuman CatV or L2Human CatK, CatL, CatC, CatX, CatHRO54441010.20.37.90.42849.0All >25,000In vitro effects of RO5444101 on recombinant cathepsin S from different species and various human proteinases. The compound showed high potency and selectivity over other cathepsins in humans. This compound also shows high potency against cathepsin S in animals.Cat, cathepsin. Open table in a new tab Table 2EC50 Values of the Cathepsin S Inhibitor RO5444101RO compoundEC50 (nmol/L)D1D2D3D4D5D6Mean ± SDRO5444101328.5174.9178.9170.9164.3118.0189.3 ± 71.7D, donor. Open table in a new tab In vitro effects of RO5444101 on recombinant cathepsin S from different species and various human proteinases. The compound showed high potency and selectivity over other cathepsins in humans. This compound also shows high potency against cathepsin S in animals. Cat, cathepsin. D, donor. Plasma levels of osteocalcin [or bone gamma-carboxyglutamate (gla) protein], osteopontin (or secreted phosphoprotein 1), and osteoprotegerin (or tumor necrosis factor receptor superfamily, member 11b) increased in mice with CRD after 5/6 nephrectomy (Table 3). Treatment of CRD mice with the cathepsin S inhibitor RO5444101 attenuated these elevations. We observed dose-dependent decreases in plasma levels of osteocalcin (P < 0.05, 6 mg/kg; P < 0.001, 60 mg/kg), osteopontin (P < 0.05, 6 mg/kg; P < 0.01, 60 mg/kg), and osteoprotegerin (P < 0.05, 6 mg/kg; P = 0.001, 60 mg/kg) compared with CRD alone (Table 3). These results indicate that RO5444101 at 60 mg/kg reduced CRD-associated elevation of these proinflammatory and pro-osteogenic markers to normal levels.Table 3Treatment with Cathepsin S Inhibitor Decreases Plasma Levels of Osteocalcin, Osteopontin, and Osteoprotegerin in Apoe−/− Mice with CRD Compared with Those without TreatmentMouse groupOsteocalcin (ng/mL)Osteopontin (ng/mL)Osteoprotegerin (ng/mL)Apoe−/−64.6 ± 28.11079.4 ± 300.11.8 ± 0.5Apoe−/− CRD161.3 ± 50.5∗P < 0.05 between the low-dose treatment group (6.6 mg/kg) and the CRD group.†P < 0.05 between the high-dose treatment group (60 mg/kg) and the CRD group.3275.1 ± 1181.0∗P < 0.05 between the low-dose treatment group (6.6 mg/kg) and the CRD group.†P < 0.05 between the high-dose treatment group (60 mg/kg) and the CRD group.2.8 ± 0.9∗P < 0.05 between the low-dose treatment group (6.6 mg/kg) and the CRD group.†P < 0.05 between the high-dose treatment group (60 mg/kg) and the CRD group.Apoe−/− CRD + 6.6 mg/kg113.6 ± 61.6∗P < 0.05 between the low-dose treatment group (6.6 mg/kg) and the CRD group.1832.7 ± 1109.0∗P < 0.05 between the low-dose treatment group (6.6 mg/kg) and the CRD group.2.2 ± 0.7∗P < 0.05 between the low-dose treatment group (6.6 mg/kg) and the CRD group.Apoe−/− CRD + 60 mg/kg96.4 ± 20.1†P < 0.05 between the high-dose treatment group (60 mg/kg) and the CRD group.1294.3 ± 478.2†P < 0.05 between the high-dose treatment group (60 mg/kg) and the CRD group.1.8 ± 0.4†P < 0.05 between the high-dose treatment group (60 mg/kg) and the CRD group.Blood samples were collected from Apoe−/− mice at the end of the treatment period, and plasma levels of the osteogenic cytokines were measured by enzyme-linked immunosorbent assay.CRD, chronic renal disease.∗ P < 0.05 between the low-dose treatment group (6.6 mg/kg) and the CRD group.† P < 0.05 between the high-dose treatment group (60 mg/kg) and the CRD group. Open table in a new tab Blood samples were collected from Apoe−/− mice at the end of the treatment period, and plasma levels of the osteogenic cytokines were measured by enzyme-linked immunosorbent assay. CRD, chronic renal disease. Intravital multichannel, high-resolution laser scanning fluorescence microscopy visualized in vivo real-time overall cathepsin activity with ProSense 750, a protease-activatable NIRF in vivo imaging agent that is activated by cathepsins, including cathepsins B, L, and S. Mice with CRD showed enhanced proteolytic signals in the carotid arteries compared with control animals, an effect that cathepsin S inhibitor treatment limited (Figure 2A). In addition, we used ex vivo macroscopic fluorescence reflectance imaging to map cathepsin activity in the aorta and carotid arteries. We observed a significant reduction in ProSense 750 signals in mice treated with RO5444101 (P < 0.01, 60 mg/kg dose versus CRD group) (Figure 2, B and C). Immunohistochemical analysis showed that cathepsin S inhibitor treatment attenuated CRD-induced accumulation of cathepsin S in the atherosclerotic plaques (Figure 2, D and E). Together, the molecular imaging and immunohistochemical analysis results suggested that cathepsin S inhibitor treatment reduced elastolytic activity and cathepsin S protein levels in the aorta of CRD mice. Cathepsin S is the most potent elastolytic enzyme. We, therefore, examined elastin fragmentation in the lesser curvature of aortic arches. We observed that high-dose RO5444101 treatment (60 mg/kg) significantly reduced the number of elastin fiber breaks, as detected by van Gieson stain (P = 0.01) (Figure 2, F and G). These results suggested that mice treated with a high dose of cathepsin S inhibitor had reduced amounts of cathepsin S and limited elastin breaks, suggesting that the specific inhibition of cathepsin S reduces elastin fragmentation. Fluorescence immunohistochemical analysis for cathepsin S, mac3, and α-smooth muscle actin in plaques from CRD mice identified the cell types responsible for cathepsin S expression (Figure 2, H and I). We found that cathepsin S localized predominantly in macrophages but that some cathepsin S expression was also observed in inflamed medial smooth muscle cells. Apoe−/− CRD mice treated with the cathepsin S inhibitor RO5444101 had decreased plaque burden in the lesser curvature of the aortic arch (P = 0.01) (Figure 3, A and D). The experiments were designed to allow the atherosclerotic lesions to develop in Apoe−/− mice on a high-fat high-cholesterol diet for 10 weeks before the induction of CRD and cathepsin S inhibition for another 10 weeks. To provide clinically relevant findings, we examined the effects of the cathepsin S–specific inhibitor RO5444101 on established atherosclerotic plaques but not on the initiation of atherosclerosis. We clearly observed significant reductions in the lesion size (Figure 3D) and macrophage accumulation (Figure 3E) in Apoe−/− CRD mice treated with a high dose of inhibitor. More importantly, th
Referência(s)