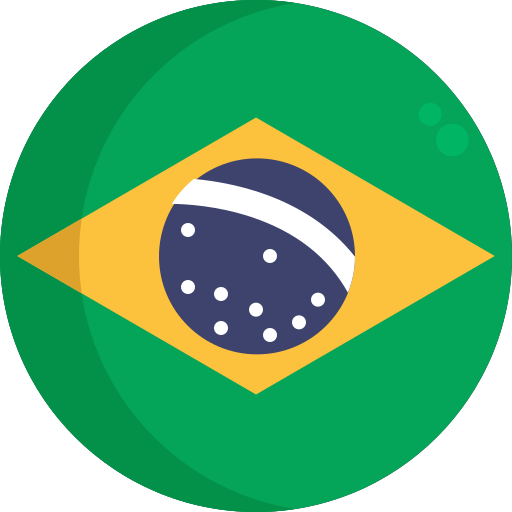
Organic Hydroperoxide Resistance Gene Encodes a Thiol-dependent Peroxidase
2003; Elsevier BV; Volume: 278; Issue: 13 Linguagem: Inglês
10.1074/jbc.m300252200
ISSN1083-351X
AutoresJosé Renato Rosa Cussiol, Simone Alves, Marcos Antônio de Oliveira, Luís Eduardo Soares Netto,
Tópico(s)Insect-Plant Interactions and Control
Resumoohr (organic hydroperoxide resistance gene) is present in several species of bacteria, and its deletion renders cells specifically sensitive to organic peroxides. The goal of this work was to determine the biochemical function of Ohr fromXylella fastidiosa. All of the Ohr homologues possess two cysteine residues, one of them located in a VCP motif, which is also present in all of the proteins from the peroxiredoxin family. Therefore, we have investigated whether Ohr possesses thiol-dependent peroxidase activity. The ohrgene from X. fastidiosa was expressed in Escherichia coli, and the recombinant Ohr decomposed hydroperoxides in a dithiothreitol-dependent manner. Ohr was about twenty times more efficient to remove organic hydroperoxides than to remove H2O2. This result is consistent with the organic hydroperoxide sensitivity of Δohr strains. The dependence of Ohr on thiol compounds was ascertained by glutamine synthetase protection assays. Approximately two thiol equivalents were consumed per peroxide removed indicating that Ohr catalyzes the following reaction: 2RSH + ROOH → RSSR + ROH + H2O. Pretreatment of Ohr with N-ethyl maleimide and substitution of cysteine residues by serines inhibited this peroxidase activity indicating that both of the Ohr cysteines are important to the decomposition of peroxides. C125S still had a residual enzymatic activity indicating that Cys-61 is directly involved in peroxide removal. Monothiol compounds do not support the peroxidase activity of Ohr as well as thioredoxin from Saccharomyces cerevisiaeand from Spirulina. Interestingly, dithiothreitol and dyhydrolipoic acid, which possess two sulfhydryl groups, do support the peroxidase activity of Ohr. Taken together our results unequivocally demonstrated that Ohr is a thiol-dependent peroxidase. ohr (organic hydroperoxide resistance gene) is present in several species of bacteria, and its deletion renders cells specifically sensitive to organic peroxides. The goal of this work was to determine the biochemical function of Ohr fromXylella fastidiosa. All of the Ohr homologues possess two cysteine residues, one of them located in a VCP motif, which is also present in all of the proteins from the peroxiredoxin family. Therefore, we have investigated whether Ohr possesses thiol-dependent peroxidase activity. The ohrgene from X. fastidiosa was expressed in Escherichia coli, and the recombinant Ohr decomposed hydroperoxides in a dithiothreitol-dependent manner. Ohr was about twenty times more efficient to remove organic hydroperoxides than to remove H2O2. This result is consistent with the organic hydroperoxide sensitivity of Δohr strains. The dependence of Ohr on thiol compounds was ascertained by glutamine synthetase protection assays. Approximately two thiol equivalents were consumed per peroxide removed indicating that Ohr catalyzes the following reaction: 2RSH + ROOH → RSSR + ROH + H2O. Pretreatment of Ohr with N-ethyl maleimide and substitution of cysteine residues by serines inhibited this peroxidase activity indicating that both of the Ohr cysteines are important to the decomposition of peroxides. C125S still had a residual enzymatic activity indicating that Cys-61 is directly involved in peroxide removal. Monothiol compounds do not support the peroxidase activity of Ohr as well as thioredoxin from Saccharomyces cerevisiaeand from Spirulina. Interestingly, dithiothreitol and dyhydrolipoic acid, which possess two sulfhydryl groups, do support the peroxidase activity of Ohr. Taken together our results unequivocally demonstrated that Ohr is a thiol-dependent peroxidase. reactive oxygen Species alkyl hydroperoxide reductase, subunit C alkyl hydroperoxide reductase, subunit F alkyl hydroperoxide reductase holo-protein dyhydrolipoic acid 5,5′-dithiobis(2-nitrobenzoic acid) dithiothreitol ferrous oxidation xylenol N-ethyl maleimide tertbutylhydroperoxide cumene hydroperoxide 2-nitro-5-thiobenzoic acid The infection of both plants and animals induces a defense response that results in an oxidative burst with the increased generation of ROS1 (1Tenhaken R. Levine A. Brisson L.F. Dixon R.A. Lamb C. Proc. Natl. Acad. Sci. U. S. A. 1995; 92: 4158-4163Google Scholar). Lipid hydroperoxides can be generated from the attack of ROS to the bacterial membrane. Organic hydroperoxides can also be formed during metabolism of certain drugs or during oxidation of n-alkanes (2Maeng J.H. Sakai Y. Tani Y. Kato N. J. Bacteriol. 1996; 178: 3695-3700Google Scholar). These peroxides can then react with metals or with metalloproteins leading to the production of secondary free radicals (3Jiang Z.-Y. Hunt J.V. Wolff S.P. Anal. Biochem. 1992; 202: 384-389Google Scholar, 4Barr D.P. Mason R.P. J. Biol. Chem. 1995; 270: 12709-12716Google Scholar), which may be related to the fact that organic peroxides possess bactericidal activity (5Akaike T. Sato K. Ijiri S. Miyamoto Y. Kohno M. Ando M. Maeda H. Arch. Biochem. Biophys. 1992; 294: 55-63Google Scholar). The alkyl hydroperoxide reductase (AhpR) is frequently considered the main enzyme responsible for the conversion of organic peroxides to the corresponding alcohols in bacteria (6Bsat N. Chen L. Helmann J.D. J. Bacteriol. 1996; 178: 6579-6586Google Scholar, 7Jacobson F.S. Morgan R.W. Christman M.F. Ames B.N. J. Biol. Chem. 1989; 264: 1488-1496Google Scholar). This enzyme comprises two subunits, AhpF and AhpC. AhpC is a thiol-dependent peroxidase that belongs to the peroxiredoxin family (8Chae H.Z. Robison K. Poole L.B. Church G. Storz G. Rhee S.G. Proc. Natl. Acad. Sci. 1994; 91: 7017-7021Google Scholar). A cysteine residue of AhpC is oxidized to sulfenic acid (R-SOH) by peroxides. NADH reduces the sulfenic acid back to its sulfhydryl (R-SH) form in a reaction catalyzed by AhpF. AhpF is a flavo-enzyme that shares homology with thioredoxin reductase (9Poole L.B. Ellis H.R. Biochemistry. 1996; 35: 56-64Google Scholar). Recently a gene was isolated in Xanthomonas campestrispv. phaseoli because its deletion rendered cells highly sensitive to killing by organic peroxides but not to H2O2 or superoxide generators (10Mongkolsuk S. Praituan W. Loprasert S. Fuangthong M. Chamnongpol S. J. Bacteriol. 1998; 180: 2636-2643Google Scholar). Therefore, it was named organic hydroperoxide resistance(ohr) gene. ohr gene expression was highly induced by t-bOOH, weakly induced by H2O2, and not induced at all by superoxide (10Mongkolsuk S. Praituan W. Loprasert S. Fuangthong M. Chamnongpol S. J. Bacteriol. 1998; 180: 2636-2643Google Scholar). Recently, homologues of this gene were also characterized in other bacteria such as Bacillus subtilis andPseudomonas aeruginosa (11Ochsner U.A. Hassett D.J. Vasil M.L. J. Bacteriol. 2001; 183: 773-778Google Scholar, 12Fuangthong M. Atichartpongkul S. Mongkolsuk S. Helmann J.D. J. Bacteriol. 2001; 183: 4134-4141Google Scholar) among others. Interestingly, Ohr, but not AhpR, appears to play a significant role in Cu-OOH resistance in B. subtilis (12Fuangthong M. Atichartpongkul S. Mongkolsuk S. Helmann J.D. J. Bacteriol. 2001; 183: 4134-4141Google Scholar). In Enterococcus faecalis, ohr deletion rendered the cells more sensitive to t-bOOH and also to ethanol (13Rince A. Giard J.C. Pichereau V. Flahaut S. Auffray Y. J. Bacteriol. 2001; 183: 1482-1488Google Scholar). Sequence analysis has shown that ohr homologues are widely spread among different bacteria genera, many of them pathogenic (14Atichartpongkul S. Loprasert S. Vattanaviboon P. Whangsuk W. Helmann J.D. Mongkolsuk S. Microbiology. 2001; 147: 1775-1782Google Scholar). Ohr also shares similarities with OsmC, which is involved in bacterial defense against osmotic stress (14Atichartpongkul S. Loprasert S. Vattanaviboon P. Whangsuk W. Helmann J.D. Mongkolsuk S. Microbiology. 2001; 147: 1775-1782Google Scholar). All the Ohr and OsmC homologues have two cysteine residues located in motifs that are also very conserved. One of the cysteine residues is part of a VCP motif that is also found in peroxiredoxins. Therefore, it was postulated that Ohr could decompose peroxides directly, similarly to AhpC, a peroxiredoxin found in bacteria (14Atichartpongkul S. Loprasert S. Vattanaviboon P. Whangsuk W. Helmann J.D. Mongkolsuk S. Microbiology. 2001; 147: 1775-1782Google Scholar). In fact, AhpC complement ohr deletion in Escherichia coli and in X. campestris (10Mongkolsuk S. Praituan W. Loprasert S. Fuangthong M. Chamnongpol S. J. Bacteriol. 1998; 180: 2636-2643Google Scholar). In P. aeruginosa, the deletion of ohr rendered the cells more sensitive to organic peroxide than ahpC deletion, and the double mutantohr, ahpC is more sensitive than the single mutants (11Ochsner U.A. Hassett D.J. Vasil M.L. J. Bacteriol. 2001; 183: 773-778Google Scholar). Finally, media from mutants for Ohr contain higher levels of organic peroxides than the correspondent wild-type cells (11Ochsner U.A. Hassett D.J. Vasil M.L. J. Bacteriol. 2001; 183: 773-778Google Scholar,15Shea R.J. Mulks M.H. Infect. Immun. 2002; 70: 794-802Google Scholar). Despite the suggestions that Ohr might directly detoxify organic hydroperoxides, it was not possible to rule out the possibility that Ohr is involved in other processes such as the transport of organic molecules (10Mongkolsuk S. Praituan W. Loprasert S. Fuangthong M. Chamnongpol S. J. Bacteriol. 1998; 180: 2636-2643Google Scholar) or in yet undefined signaling pathways that lead to activation of secondary molecules that would then inactivate organic peroxides. Here, for the first time, the biochemical activity of Ohr was elucidated: Ohr from Xylella fastidiosa possesses a thiol-dependent peroxidase activity, which is probably responsible for the hypersensitivity of Δohr mutants to treatment with organic hydroperoxides. All the reagents were purchased with the highest degree of purity. DHLA was purchased in the reduced form from Sigma (T8620). DHLA is a yellow oil, and its stock solution was prepared by dilution to 50 mm concentration in 20 mm phosphate buffer, pH = 7.5 and heated at 45 °C for 30 min. DHLA concentration was ascertained by the use of the Ellman's reagent as described bellow. The ohr gene was PCR-amplified from the cosmid XF-07F02 that was used in the X. fastidiosasequencing genome project (16Simpson A.J.G. Reinach F.C. Arruda P. Abreu F.A. Acencio M. Alvarenga R. Alves L.M. Araya J.E. Baia G.S. Baptista C.S. Barros M.H. Bonaccorsi E.D. Bordin S. Bove J.M. Briones M.R. Bueno M.R. Camargo A.A. Camargo L.E. Carraro D.M. Carrer H. Colauto N.B. Colombo C. Costa F.F. Costa M.C. Costa-Neto C.M. Coutinho L.L. Cristofani M. Dias-Neto E. Docena C. El-Dorry H. Facincani A.P. Ferreira A.J. Ferreira V.C. Ferro J.A. Fraga J.S. Franca S.C. Franco M.C. Frohme M. Furlan L.R. Garnier M. Goldman G.H. Goldman M.H. Gomes S.L. Gruber A. Ho P.L. Hoheisel J.D. Junqueira M.L. Kemper E.L. Kitajima J.P. Krieger J.E. Kuramae E.E. Laigret F. Lambais M.R. Leite L.C.C. Lemos E.G. Lemos M.V. Lopes S.A. Lopes C.R. Machado J.A. Machado M.A. Madeira A.M. Madeira H.M. Marino C.L. Marques M.V. Martins E.A. Martins E.M. Matsukuma A.Y. Menck C.F. Miracca E.C. Miyaki C.Y. Monteiro-Vitorello C.B. Moon D.H. Nagai M.A. Nascimento A.L. Netto L.E.S. Nhani Jr., A. Nobrega F.G. Nunes L.R. Oliveira M.A. de Oliveira M.C. de Oliveira R.C. Palmieri D.A. Paris A. Peixoto B.R. Pereira G.A.G. Pereira Jr., H.A. Pesquero J.B. Quaggio R.B. Roberto P.G. Rodrigues V. de M. Rosa A.J. de Rosa Jr., V.E. de Sa R.G. Santelli R.V. Sawasaki H.E. da Silva A.C. da Silva A.M. da Silva F.R. da Silva Jr., W.A. da Silveira J.F. Silvestri M.L. Siqueira W.J. de Souza A.A. de Souza A.P. Terenzi M.F. Truffi D. Tsai S.M. Tsuhako M.H. Vallada H. Van Sluys M.A. Verjovski-Almeida S. Vettore A.L. Zago M.A. Zatz M. Meidanis J. Setubal J.C. Nature. 2000; 406: 151-159Google Scholar). The following forward 5′-CGCGGATCCCATATGAATTCACTGGAG (Xfo1) and reverse 5′-CGCAAGCTTGGATCCTTAGTCAATCAG (Xfo2) primers were used. The underlined bases represent the NdeI and BamHI sites, respectively. The PCR product was cloned into the pGEM-T easy vector (Promega) resulting in the pGEM/ohr plasmid. AnE. coli DH5-α strain was transformed with pGEM/ohr, and white colonies were selected from LB-ampicillin-5-bromo-4-chloro-3-indolyl-β-d-galactopyranoside (X-gal) medium. Plasmid extraction was performed using the Rapid Plasmid Miniprep System Concert kit (Invitrogen). The plasmid PGEM/ohr was used to generate the two individually ohr mutant proteins, C61S and C125S, in which cysteines Cys-61 and Cys-125 were replaced by serines through PCR megaprimer methods (17Sarkar G. Sommer S.S. BioTechniques. 1990; 4: 404-407Google Scholar, 18Datta A.K. Nucleic Acids Res. 1995; 21: 4530-4531Google Scholar). In the case of the C61S construct, PCR was performed first with the mutagenic primer XfoC1 forward 5′-TTATTCTGCCTCTTTCATTGG-3′ and Xfo2 reverse, where the bold letters denote the mutation performed. A single band of 277 bp was eluted out from the agarose gel and used as a primer (megaprimer) along with the primer Xfo1 to the second PCR step to amplify the rest of Ohr gene. In the case of the C125S construct, replacement was performed in one-step PCR using the primers Xfo1 and a large terminal mutagenic primer XfoC2 5′-CGCAAGCTTGGATCCTTAGTCAATCAGAATCAAAACGACGTCGATATTTCCACGGGTTGCATTAGAGTACGGAGAAACACGATGC-3′, where the boldface typing represents the codon mutation and the underlined bases represents the BamHI restriction site. The final mutated PCR products were ligated in pGEMT easy vector to produce PGEM/C61S and PGEM/C125S, independently. pET15b, pGEM/ohr, PGEM/ohrC61S, and PGEM/C125S were first digested with NdeI and after by BamHI. The fragments generated byNdeI/BamHI digestion of plasmids derived from pGEMs were extracted from agarose gel by the Rapid Gel Extraction Concert kit (Invitrogen) and were individually ligated to the digested pET-15b expression vector. The resulting pET15b/ohr, PET15b/C61S, and PET15b/C125S plasmids were sequenced in an Applied Biosystems ABI Prism 377 96 to confirm that the constructions were correct. An E. coli DH5-α strain was transformed with the expression vectors and cultured to increase plasmid production. Another plasmid extraction was performed, andE. coli Bl21(DE3) cells were transformed with the same constructs. The resulting strains were used for expression and purification of Ohr, C61S, and C125S. E. coliBl21(DE3) strains transformed with pET15b/ohr, pET15b/C61S, and pET15b/C125S were cultured (50 ml) overnight in LB + ampicillin medium, transferred to 1 liter of fresh LB + ampicillin (100 μg/ml) medium, and cultured further until the A 600 reached 0.6–0.8. Isopropyl-1-thio-β-d-galactopyranoside was then added to a final concentration equivalent to 1 mm. After 3 h of incubation, cells were harvested by centrifugation. The pellet was washed and suspended in the start buffer (phosphate buffer, 20 mm, pH 7.4). Seven cycles of 30 s of sonication (35% amplitude) following 30 s in ice were applied to cell suspension. The cell extracts were kept in ice during streptomycin sulfate 1% treatment for 15 min. The suspension was centrifuged at 31,500 × g for 30 min to remove nucleic acid precipitates. Finally cell extract was applied to a nickel affinity column (Hi-trap from Amersham Biosciences). The conditions of protein purification were optimized using the gradient procedure for imidazole concentration described by the manufacturer. Peroxide concentration was determined by the ferrous oxidation xylenol (FOX) assay as previously described (3Jiang Z.-Y. Hunt J.V. Wolff S.P. Anal. Biochem. 1992; 202: 384-389Google Scholar). Reactions were initiated by the addition of thiol compounds and stopped at different intervals by addition of 20 μl of HCl (1m) into 100-μl reaction mixtures. No peroxide consumption was detected in the absence of thiols. H2O2 concentration in stock solutions was checked by its absorbance (ε240 nm = 43.6m−1·cm−1). The amount of thiol groups remaining in solution was determined by the Ellman's reagent (DTNB), using the ε412 nm = 13,600m−1·cm−1 for 2-nitro-5-thiobenzoic acid (TNB) (19Riddles P.W. Robert L.B. Zerner B. Methods Enzymol. 1983; 91: 49-60Google Scholar). As described above, reactions were stopped at different intervals by addition of 20 μl of HCl (1m) into 100-μl reaction mixtures. Samples were neutralized by dilution (1:10) in a solution containing Hepes (1m, pH 7.4) and DTNB (5 mm). Absorbance at 412 nm was immediately recorded. Antioxidant activities of Ohr, OhrC61S, OhrC125S, and cTPxI were measured by their ability to protect glutamine synthetase from oxidative inactivation. Several H2O2-removing enzymes such as GSH peroxidase, catalase, and cTPxI can protect glutamine synthetase (20Kim K. Kim I.H. Lee Ki-Y. Rhee S.G. Stadtman E.R. J. Biol. Chem. 1988; 263: 4704-4711Google Scholar). The procedure used to determine glutamine synthetase activity was the same described by Kim et al. (20Kim K. Kim I.H. Lee Ki-Y. Rhee S.G. Stadtman E.R. J. Biol. Chem. 1988; 263: 4704-4711Google Scholar). Recombinant Ohr (2 mg/ml) was treated with NEM (1 mm) for 1 h at room temperature and then was dialyzed against phosphate buffer (20 mm), pH 7.4. The concentration of histidine-tagged Ohr was determined using the extinction coefficient ε280 nm = 3960 m−1·cm−1, which was determined using the software of ExPASy proteomics from Swiss Institute of bioinformatics (ca.expasy.org/tools/protparam.html). Histidine tag of recombinant Ohr was digested with thrombin (0.01 units/μl) using the thrombin cleavage capture kit from Novagen. The digestion was carried out for 16 h at 25 °C. The concentration of the recombinant protein without histidine tag was determined spectrophotometrically, using the same extinction coefficient ε280 nm = 3960m−1·cm−1 determined above because the histidine tag does not contain optically active residues. Determination of sulfenic acid (R-SOH) in wild-type as well as in mutant proteins was performed by the TNB anion method described by Ellis and Poole (21Ellis H.R. Poole L.B. Biochemistry. 1997; 36: 13349-13356Google Scholar). In summary, TNB was prepared by incubation of an almost equimolar mixture of DTNB and DTT (1 DTNB:0.9 SH). Proteins preincubated or not with peroxides were treated with a 20-fold excess of TNB. As described before (21Ellis H.R. Poole L.B. Biochemistry. 1997; 36: 13349-13356Google Scholar), TNB reacts with sulfenic acids in a 1:1 stoichiometry, generating a mixed disulfide between TNB and a cysteine residue. Excess of TNB was removed by PD-10 desalting column (Amersham Biosciences). Mixed disulfides were then treated with 10-fold excess of DTT, and the amount TNB released (which was equal to the amount sulfenic acid formed) was determined spectrophotometrically. Ohr from X. fastidiosa possesses a very high degree of similarity with proteins from various bacteria such as X. campestris pv.phaseoli and P. aeruginosa (14Atichartpongkul S. Loprasert S. Vattanaviboon P. Whangsuk W. Helmann J.D. Mongkolsuk S. Microbiology. 2001; 147: 1775-1782Google Scholar). In general, theohr gene is present in a single copy, but in some cases such as B. subtilis, Mesorhizobium loti, andRalstonia solanacearum two copies of ohr are present (12Fuangthong M. Atichartpongkul S. Mongkolsuk S. Helmann J.D. J. Bacteriol. 2001; 183: 4134-4141Google Scholar, 22Kaneko T. Nakamura Y. Sato S. Asamizu E. Kato T. Sasamoto S. Watanabe A. Idesawa K. Ishikawa A. Kawashima K. Kimura T. Kishida Y. Kiyokawa C. Kohara M. Matsumoto M. Matsuno A. Mochizuki Y. Nakayama S. Nakazaki N. Shimpo S. Sugimoto M. Takeuchi C. Yamada M. Tabata S. DNA Res. 2000; 7: 331-338Google Scholar, 23Salanoubat M. Genin S. Artiguenave F. Gouzy J. Mangenot S. Arlat M. Billault A. Brottier P. Camus J.C. Cattolico L. Chandler M. Choisne N. Claudel-Renard C. Cunnac S. Demange N. Gaspin C. Lavie M. Moisan A. Robert C. Saurin W. Schiex T. Siguier P. Thebault P. Whalen M. Wincker P. Levy M. Weissenbach J. Boucher C.A. Nature. 2002; 415: 497-502Google Scholar). In Streptomyces coelicolor, three copies of ohr appear to be present (24Redenbach M. Kieser H.M. Denapaite D. Eichner A. Cullum J. Kinashi H. Hopwood D.A. Mol. Microbiol. 1996; 21: 77-96Google Scholar). A blastp analysis on theX. fastidiosa genome using the tools available at the site aeg.lbi.ic.unicamp.br/xf/detected only one copy of ohr in this bacteria located between coordinates 1,742,868 and 1,743,299 with 432 nucleotides. The predicted amino acid sequence possesses 143 residues and a molecular mass equivalent to 14.9 kDa. Among other characteristics Ohr proteins have two conserved cysteine residues that are at positions 61 and 125 in the homologue from X. fastidiosa. Based on sequence homology two domains of Ohr can be defined: domain 1, which contains cysteine 61 and a high number of hydrophobic residues, and domain 2, which has cysteine 125 in a VCP motif (Fig. 1 A). The VCP motif is also present in the peroxiredoxin family, whose proteins are thiol-dependent peroxidases (25Rhee S.G. Kang S.W. Netto L.E.S. Seo M.S. Stadtman E.R. Biofactors. 1999; 10: 207-209Google Scholar). The two proposed domains are highly conserved among Ohr homologues, especially domain 1 (14Atichartpongkul S. Loprasert S. Vattanaviboon P. Whangsuk W. Helmann J.D. Mongkolsuk S. Microbiology. 2001; 147: 1775-1782Google Scholar) (Fig. 1 A). Because ohr deletion renders cells very sensitive to organic peroxide treatment, a hydrophobicity analysis of Ohr protein was carried out (26Kyte J. Doolittle R.F. J. Mol. Biol. 1982; 157: 105-132Google Scholar). Our data indicated that cysteine 61 is in a highly hydrophobic environment, whereas cysteine 125 is in a hydrophilic environment (Fig.1 B). To investigate whether Ohr possesses thiol-dependent peroxidase activity, ohr gene from X. fastidiosawas expressed in E. coli and purified by nickel affinity chromatography (see "Materials and Methods"). A histidine tag recombinant Ohr was obtained with high degree of purity as ascertained by SDS-PAGE (Fig. 2). Two bands corresponding to Ohr were observed, both of which migrate closely to 17 kDa, as expected for a monomer (Fig. 2 A). Removal of histidine tag by thrombin proteolysis also resulted in two bands both of which migrated at ∼15 kDa (data not shown). The abundance of each band was dependent on the oxidative condition to which Ohr was exposed (Fig. 2) and was independent of the presence of the histidine tag (data not shown). Bands corresponding to dimers did not appear even after treatments of Ohr with H2O2 or organic hydroperoxide at various concentrations (Fig. 2 B) indicating that no disulfide bond was formed between two Ohr molecules. Since the two bands observed in Fig. 2 have approximately the monomer size, they should represent different configurations of Ohr monomers. The lower band (band a) should correspond to an oxidized state of Ohr, which is generated after H2O2treatment or at mild concentrations of t-bOOH (Fig.2 B). On the other side, the upper band (band b) may represent a mixture of reduced and oxidized states of Ohr. This is because Ohr treatment with increasing concentrations of DTT provoked augment of band b intensity (Fig.2 A) as well as Ohr treatment with high organic peroxide concentrations (Fig. 2 B). The meanings of these two bands are further discussed below, after the description of results with Ohr site-specific mutants. In any case, it is important to emphasize that different monomeric configurations were also observed before for cTPxI, a thiol-dependent peroxidase from Saccharomyces cerevisiae (27Chae H.Z. Uhm T.B. Rhee S.G. Proc. Natl. Acad. Sci. (U. S. A.). 1994; 91: 7022-7026Google Scholar). Recombinant Ohr decomposed peroxides only if DTT was also present in the reaction mixture (Fig. 3). Because the substrates were present in a 350–4100-excess over the protein, it is reasonable to think that Ohr was acting catalytically. In the conditions described in Fig. 3, the specific activity of Ohr was 20.0, 17.0, and 1.3 μm/min/ng when, respectively,t-bOOH, Cu-OOH, and H2O2 were considered the substrates. This indicates that Ohr was 10–20 times more efficient in the removal of organic peroxides than in the removal of H2O2. These results are consistent with the high susceptibility of Δohr mutants to organic peroxides but not to H2O2 (Ref. 14Atichartpongkul S. Loprasert S. Vattanaviboon P. Whangsuk W. Helmann J.D. Mongkolsuk S. Microbiology. 2001; 147: 1775-1782Google Scholar and references cited herein). In any case, Ohr did decompose H2O2when DTT was present in the reaction mixture. This ability of Ohr to decompose peroxides was dependent on the integrity of its cysteine residues because pretreatment of Ohr with NEM inhibited its peroxidase activity (Fig. 3 C). Therefore, it is reasonable to think that Cys-61, Cys-125, or both are directly involved in the decomposition of peroxides by Ohr. Ohr also possesses antioxidant property as demonstrated by its capacity to protect glutamine synthetase from inactivation by thiol-containing oxidative system composed of DTT/Fe3+/O2 or composed of DHLA/Fe3+/O2 (Fig.4 A). Probably, this protection was due to the thiol-dependent peroxidase activity of Ohr because other thiol-dependent peroxidase such as GSH peroxidase and cTPxI also protect glutamine synthetase from inactivation (20Kim K. Kim I.H. Lee Ki-Y. Rhee S.G. Stadtman E.R. J. Biol. Chem. 1988; 263: 4704-4711Google Scholar). In fact, the glutamine synthetase protection assay has been used to investigate whether proteins possess peroxide-removing activity (28Lee S.P. Hwang Y.S. Kim Y.J. Kwon K.S. Kim H.J. Kim K. Chae H.Z. J. Biol. Chem. 2001; 276: 29826-29832Google Scholar, 29Yamashita H. Avraham S. Jiang S. London R. Van Veldhoven P.P. Subramani S. Rogers R.A. Avraham H. J. Biol. Chem. 1999; 274: 29897-29904Google Scholar). Ohr did not protect glutamine synthetase against the ascorbate/Fe3+/O2 system, indicating that this protein is a thiol-specific antioxidant protein (Fig. 4 A). The protective activity of Ohr was dependent on the concentration of protein and is comparable to the cTPxI activity (Fig. 4 B). Removal of histidine tag by thrombin treatment did not alter the enzymatic characteristics of Ohr nor its ability to protect glutamine synthetase (data not shown). When H2O2 was used as substrate, the specific activity of Ohr (Fig. 3) was similar to cTPxI (data not shown). These results are consistent with the glutamine synthetase assays (Fig.4 B) where these two proteins were also equally protective. The oxidative inactivation of glutamine synthetase is dependent on H2O2 formation by metal catalyzed oxidation systems and by its posterior conversion to hydroxyl radical through the Fenton reaction (30Stadtman E.R. Free Rad. Biol. Med. 1990; 9: 315-325Google Scholar). Therefore protection of glutamine synthetase from inactivation probably occurs through the removal of H2O2 by antioxidant enzymes (31Netto L.E.S. Chae H.Z. Kang S.W. Rhee S.G. Stadtman E.R. J. Biol. Chem. 1996; 271: 15315-15321Google Scholar). The role of Ohr cysteines in peroxide reduction was strongly suggested since treatment of Ohr with NEM lead to protein inactivation (Fig. 3). To specifically investigate the roles of Cys-61 and Cys-125 on Ohr catalysis these two residues were individually replaced by serine generating, respectively, C61S and C125S. Initially, the capacity of the mutant proteins to decompose hydroperoxides was investigated. C61S and C125S had no detectable peroxidase activity when t-bOOH was used as a substrate (Fig.5 A). When H2O2 was the substrate, C125S had a residual activity, whereas C61S did not decompose any peroxide (Fig.5 B). These results indicated that both cysteine residues are important for catalysis. Replacement of either Cys-61 or Cys-125 also provoked great decreases in the ability of Ohr to protect glutamine synthetase from oxidative inactivation (Fig. 5 C). C61S only showed some protective effect at very high doses, which might be attributable to nonspecific activity. Interestingly, replacement of two of the cysteines of human peroxiredoxin V (prx V), which forms a stable intramolecular disulfide intermediate during its catalytic cycle, produced similar effects (32Seo M.S. Kang S.W. Kim K. Baines I.C. Lee T.H. Rhee S.G. J. Biol. Chem. 2000; 275: 20346-20354Google Scholar). Therefore, the results described in Fig.5 represented an initial suggestion that an intramolecular disulfide is also a reaction intermediate of wild-type Ohr. Further evidences are presented below. The migration of Ohr mutants was also analyzed by SDS-PAGE (Fig.6) under both reducing and non-reducing conditions to understand the meaning of the two monomeric bands observed in Fig. 2. As standards for mutant proteins, wild-type Ohr was treated with peroxides (60 μm) and with DTT (100 mm), which provoked the appearance of the monomeric lower (band a) and upper band (band b) respectively. Interestingly, neither C61S nor C125S, in any condition tested, migrated at the same position that the wild-type protein treated with peroxides (60 μm) (band a) (Fig. 6). Therefore, band aobserved for the wild-type protein (Fig. 2) should represent an intramolecular intermediate since any of the mutants can form an intramolecular disulfide bond. This was expected since an intramolecular disulfide bond should lead to a more compact protein configuration that would migrate faster than the other REDOX states. Interestingly, two other bands, named c and d, could also be observed in C125S exposed to oxidative conditions (Fig.6 A). Band d was detected in non-treated C125S (data not shown) as well as in C125S submitted to mild oxidative conditions (Fig. 6 A, lane 7). Under stronger oxidative conditions, band c became predominant and band d disappeared (Fig. 6 A,lane 6 and data not shown). Sometimes a band of ∼30 kDa was observed in substitution of band c after C125S treatment with organic peroxides (data not shown). The meaning ofband c is unknown and could be attribu
Referência(s)