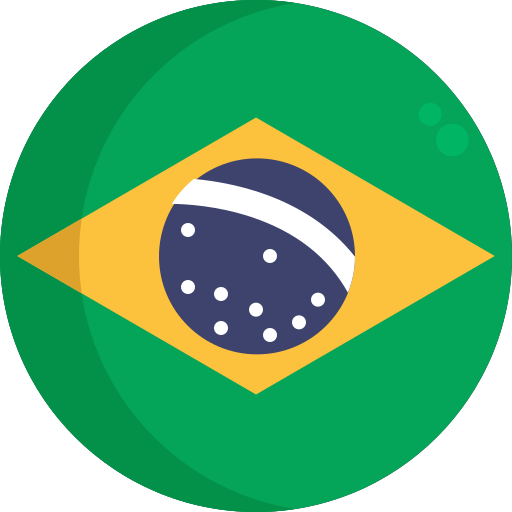
Structural Basis for Metal Ion Coordination and the Catalytic Mechanism of Sphingomyelinases D
2005; Elsevier BV; Volume: 280; Issue: 14 Linguagem: Inglês
10.1074/jbc.m412437200
ISSN1083-351X
AutoresM.T. Murakami, Matheus de Freitas Fernandes‐Pedrosa, Denise V. Tambourgi, Raghuvir K. Arni,
Tópico(s)Endoplasmic Reticulum Stress and Disease
ResumoSphingomyelinases D (SMases D) from Loxosceles spider venom are the principal toxins responsible for the manifestation of dermonecrosis, intravascular hemolysis, and acute renal failure, which can result in death. These enzymes catalyze the hydrolysis of sphingomyelin, resulting in the formation of ceramide 1-phosphate and choline or the hydrolysis of lysophosphatidyl choline, generating the lipid mediator lysophosphatidic acid. This report represents the first crystal structure of a member of the sphingomyelinase D family from Loxosceles laeta (SMase I), which has been determined at 1.75-Å resolution using the "quick cryo-soaking" technique and phases obtained from a single iodine derivative and data collected from a conventional rotating anode x-ray source. SMase I folds as an (α/β)8 barrel, the interfacial and catalytic sites encompass hydrophobic loops and a negatively charged surface. Substrate binding and/or the transition state are stabilized by a Mg2+ ion, which is coordinated by Glu32, Asp34, Asp91, and solvent molecules. In the proposed acid base catalytic mechanism, His12 and His47 play key roles and are supported by a network of hydrogen bonds between Asp34, Asp52, Trp230, Asp233, and Asn252. Sphingomyelinases D (SMases D) from Loxosceles spider venom are the principal toxins responsible for the manifestation of dermonecrosis, intravascular hemolysis, and acute renal failure, which can result in death. These enzymes catalyze the hydrolysis of sphingomyelin, resulting in the formation of ceramide 1-phosphate and choline or the hydrolysis of lysophosphatidyl choline, generating the lipid mediator lysophosphatidic acid. This report represents the first crystal structure of a member of the sphingomyelinase D family from Loxosceles laeta (SMase I), which has been determined at 1.75-Å resolution using the "quick cryo-soaking" technique and phases obtained from a single iodine derivative and data collected from a conventional rotating anode x-ray source. SMase I folds as an (α/β)8 barrel, the interfacial and catalytic sites encompass hydrophobic loops and a negatively charged surface. Substrate binding and/or the transition state are stabilized by a Mg2+ ion, which is coordinated by Glu32, Asp34, Asp91, and solvent molecules. In the proposed acid base catalytic mechanism, His12 and His47 play key roles and are supported by a network of hydrogen bonds between Asp34, Asp52, Trp230, Asp233, and Asn252. Envenomation by arachnids of the genus Loxosceles (brown spider), endemic to temperate and tropical regions of the Americas, Africa, and Europe, leads to local dermonecrosis and also to serious systemic toxicity. Three principal Loxosceles species of medical importance are encountered in Brazil (Loxosceles laeta, Loxosceles intermedia, Loxosceles gaucho), and more than 2,000 cases of envenomation by L. intermedia alone are reported each year. In the United States, six Loxosceles species (including Loxosceles reclusa, brown recluse) are responsible for numerous incidents (1.Futrell J.M. Am. J. Med. Sci. 1992; 304: 261-267Crossref PubMed Scopus (245) Google Scholar). L. laeta, possibly the most toxic and dangerous of all the species, is widely distributed and is encountered as far north as Canada (2.Nelson J. Can. Med. Assoc. J. 1988; 138: 888-889Google Scholar, 3.Allen P.B. Can. Med. Assoc. J. 1988; 138: 792Google Scholar) and is endemic primarily in South and Central America.The site of envenomation, which initially causes only minor discomfort, begins as an expanding area of erythema and edema. A centrally located necrotic ulcer often forms 8–24 h after envenomation (4.Atkins J.A. Wingo C.W. Sodeman W.A. Flynn J.E. Am. J. Trop. Med. Hyg. 1958; 7: 165-184Crossref PubMed Scopus (96) Google Scholar, 5.Wasserman G.S. Anderson D.O. J. Toxicol. Clin. Toxicol. 1983; 21: 451-455Crossref PubMed Scopus (102) Google Scholar). Extensive tissue destruction follows, with the ulcer taking many months to heal, and in extreme cases requires debridement or skin grafting. The lesions are remarkable considering that Loxosceles spiders inject only a few tenths of a microliter of venom containing no more than 30 μg of protein.Mild systemic effects induced by envenomation, such as fever, malaise, pruritus, and exanthema are common, whereas intravascular hemolysis and coagulation, sometimes accompanied by thrombocytopenia and renal failure, occur in ∼16% of the victims (1.Futrell J.M. Am. J. Med. Sci. 1992; 304: 261-267Crossref PubMed Scopus (245) Google Scholar, 6.Barreto O.C. Cardoso J.L. De Cillo D. Rev. Inst. Med. Trop. São Paulo. 1985; 27: 264-267Crossref PubMed Scopus (35) Google Scholar, 7.Schenone H. Saavedra T. Rojas A. Villarroel F. Rev. Inst. Med. Trop. São Paulo. 1989; 31: 403-415Crossref PubMed Scopus (73) Google Scholar, 8.Ginsburg C.M. Weinberg A.G. J. Pediatr. 1988; 112: 496-499Abstract Full Text PDF PubMed Scopus (18) Google Scholar, 9.Sezerino U.M. Zannin M. Coelho L.K. Gonçalves Jr., J. Grando M. Mattosinho S.G. Cardoso J.L. von Eickstedt V.R. França F.O. Barbaro K.C. Fan H.W. Trans. R. Soc. Trop. Med. Hyg. 1998; 92: 546-548Abstract Full Text PDF PubMed Scopus (97) Google Scholar, 10.Gendron B.P. Am. J. Emerg. Med. 1990; 8: 51-54Abstract Full Text PDF PubMed Scopus (35) Google Scholar, 11.Bey T.A. Walter F.G. Lober W. Schmidt J. Spark R. Schlievert P.M. Ann. Emerg. Med. 1997; 30: 701-703Abstract Full Text Full Text PDF PubMed Scopus (31) Google Scholar). Although systemic loxoscelism is less common than the cutaneous form, it is the main cause of death associated with Loxosceles envenomation. Most of the deaths occur in children and are related to the South American species L. laeta (1.Futrell J.M. Am. J. Med. Sci. 1992; 304: 261-267Crossref PubMed Scopus (245) Google Scholar). Due to our limited understanding of the venom's mechanism of action, effective treatment is currently not available.The recombinant sphingomyelinases (SMases) 1The abbreviations used are: SMase, sphingomyelinase; SM, sphingomyelin; PLD, phospholipase D. 1The abbreviations used are: SMase, sphingomyelinase; SM, sphingomyelin; PLD, phospholipase D. D of L. laeta and L. intermedia retain all the local and systemic effects observed in the whole venom, inducing dermonecrosis in rabbits and rendering human erythrocytes susceptible to lysis by complement (12.Tambourgi D.V. Magnoli F.C. Von Eickstedt V.R. Benedetti Z.C. Petricevich V.L. da Silva W.D. J. Immunol. 1995; 155: 4459-4466PubMed Google Scholar, 13.Tambourgi D.V. Magnoli F.C. van den Berg C.W. Morgan B.P. de Araujo P.S. Alves E.W. da Silva W.D. Biochem. Biophys. Res. Commun. 1998; 251 (W.): 366-373CCrossref PubMed Scopus (138) Google Scholar, 14.Tambourgi D.V. Fernandes-Pedrosa M.F. van den Berg C.W. Gonçalves-de-Andrade R.M. Ferracini M. Paixão-Cavalcante D. Morgan B.P. Rushmere N.K. Mol. Immunol. 2004; 41 (F.): 831-840MCrossref PubMed Scopus (61) Google Scholar, 15.Fernandes-Pedrosa M.F. Junqueira de Azevedo I.L. Gonçalves-de-Andrade R.M. van den Berg C.W. Ramos C.R. Ho P.L. Tambourgi D.V. Biochem. Biophys. Res. Commun. 2002; 298: 638-645Crossref PubMed Scopus (96) Google Scholar). In a mouse model of Loxosceles envenomation, they also induce intravascular hemolysis and provoke a cytokine response, which resembles that observed in endotoxic shock (16.Tambourgi D.V. Petricevich V.L. Magnoli F.C. Assaf S.L. Jancar S. Da Silva W.D. Toxicon. 1998; 36: 391-403Crossref PubMed Scopus (62) Google Scholar). SMases facilitate activation of the alternative pathway of complement on human erythrocytes by removal of glycophorins as a consequence of the activation of an endogenous metalloproteinase (17.Tambourgi D.V. Morgan B.P. Gonçalves de Andrade R.M. Magnoli F.C. van den Berg C.W. Blood. 2000; 95: 683-691Crossref PubMed Google Scholar) and activation of the classical pathway of complement, possibly by disruption of the membrane asymmetry (18.Tambourgi D.V. Silva M.S. Billington S.J. Gonçalves de Andrade R.M. Magnoli F.C. Songer J.G. van den Berg C.W. Immunology. 2002; 107: 93-101Crossref PubMed Scopus (71) Google Scholar). SMases D are not encountered elsewhere in the animal kingdom; however, a similar enzyme is produced as an exotoxin by some pathogenic bacteria, notably Corynebacterium pseudotuberculosis, Corynebacterium ulcerans, and Arcanobacterium (formerly Corynebacterium) hemolyticum (19.Soucek A. Michalec C. Souckova A. Biochim. Biophys. Acta. 1967; 144: 180-182Crossref PubMed Scopus (28) Google Scholar, 20.Truett A.P. King Jr., L.E. Adv. Lipid Res. 1993; 26: 275-291PubMed Google Scholar, 21.McNamara P.J. Cuevas W.A. Songer J.G. Gene. 1995; 156: 113-118Crossref PubMed Scopus (81) Google Scholar). C. pseudotuberculosis causes lymphadenitis in animals and is also pathogenic to humans, whereas C. ulcerans and A. hemolyticum are pathogens of pharyngitis and other human infections (22.Songer J.G. Trends Microbiol. 1997; 5: 156-161Abstract Full Text PDF PubMed Scopus (196) Google Scholar). The SMase D from C. pseudotuberculosis, also named sphingomyelin (SM)-specific phospholipase D (PLD), is an essential virulence determinant that contributes to the persistence and spread of the bacteria within the host (23.McNamara P.J. Bradley G.A. Songer J.G. Mol. Microbiol. 1994; 12: 921-930Crossref PubMed Scopus (87) Google Scholar).The Loxosceles and bacterial SMases D possess similar molecular masses (31–35 kDa) but share only limited sequence homology (13.Tambourgi D.V. Magnoli F.C. van den Berg C.W. Morgan B.P. de Araujo P.S. Alves E.W. da Silva W.D. Biochem. Biophys. Res. Commun. 1998; 251 (W.): 366-373CCrossref PubMed Scopus (138) Google Scholar, 24.van Meeteren L.A. Frederiks F. Giepmans B.N. Fernandes-Pedrosa M.F. Billington S.J. Jost B.H. Tambourgi D.V. Moolenaar W.H. J. Biol. Chem. 2004; 279: 10833-10836Abstract Full Text Full Text PDF PubMed Scopus (109) Google Scholar). In model systems, the Loxosceles and C. pseudotuberculosis enzymes provoke similar pathophysiological effects, including platelet aggregation, endothelial hyperpermeability, complement-dependent hemolysis, and neutrophil recruitment (13.Tambourgi D.V. Magnoli F.C. van den Berg C.W. Morgan B.P. de Araujo P.S. Alves E.W. da Silva W.D. Biochem. Biophys. Res. Commun. 1998; 251 (W.): 366-373CCrossref PubMed Scopus (138) Google Scholar, 14.Tambourgi D.V. Fernandes-Pedrosa M.F. van den Berg C.W. Gonçalves-de-Andrade R.M. Ferracini M. Paixão-Cavalcante D. Morgan B.P. Rushmere N.K. Mol. Immunol. 2004; 41 (F.): 831-840MCrossref PubMed Scopus (61) Google Scholar, 15.Fernandes-Pedrosa M.F. Junqueira de Azevedo I.L. Gonçalves-de-Andrade R.M. van den Berg C.W. Ramos C.R. Ho P.L. Tambourgi D.V. Biochem. Biophys. Res. Commun. 2002; 298: 638-645Crossref PubMed Scopus (96) Google Scholar, 25.Forrester L.J. Barrett J.T. Campbell B.J. Arch. Biochem. Biophys. 1978; 187: 355-365Crossref PubMed Scopus (121) Google Scholar, 26.Kurpiewski G. Forrester L.J. Barrett J.T. Campbell B.J. Biochim. Biophys. Acta. 1981; 678: 467-476Crossref PubMed Scopus (135) Google Scholar, 27.Carne H.R. Onon E.O. Nature. 1978; 271: 246-248Crossref PubMed Scopus (38) Google Scholar, 28.Bernheimer A.W. Campbell B.J. Forrester L.J. Science. 1985; 228: 590-591Crossref PubMed Scopus (49) Google Scholar).Of the four major phospholipids present in the outer leaflet of the mammalian plasma membranes, only sphingomyelin is hydrolyzed by bacterial PLD and spider toxins, resulting in the formation of ceramide-1-phosphate (Cer-1-P or N-acyl-sphingosine-1-phosphate) (13.Tambourgi D.V. Magnoli F.C. van den Berg C.W. Morgan B.P. de Araujo P.S. Alves E.W. da Silva W.D. Biochem. Biophys. Res. Commun. 1998; 251 (W.): 366-373CCrossref PubMed Scopus (138) Google Scholar, 25.Forrester L.J. Barrett J.T. Campbell B.J. Arch. Biochem. Biophys. 1978; 187: 355-365Crossref PubMed Scopus (121) Google Scholar, 26.Kurpiewski G. Forrester L.J. Barrett J.T. Campbell B.J. Biochim. Biophys. Acta. 1981; 678: 467-476Crossref PubMed Scopus (135) Google Scholar).In the presence of Mg2+, spider and bacterial SMases D catalyze the release of choline from lysophosphatidylcholine but not from phosphatidylcholine (24.van Meeteren L.A. Frederiks F. Giepmans B.N. Fernandes-Pedrosa M.F. Billington S.J. Jost B.H. Tambourgi D.V. Moolenaar W.H. J. Biol. Chem. 2004; 279: 10833-10836Abstract Full Text Full Text PDF PubMed Scopus (109) Google Scholar). Plasma lysophosphatidylcholine is tightly bound to albumin, and removal of its choline headgroup yields lysophosphatidic acid, a potent lipid mediator with numerous biological activities in many different cell types (29.Moolenaar W.H. Exp. Cell Res. 1999; 253: 230-238Crossref PubMed Scopus (371) Google Scholar, 30.Chun J. Goetzl E.J. Hla T. Igarashi Y. Lynch K.R. Moolenaar W. Pyne S. Tigyi G. Pharmacol. Rev. 2002; 54: 265-269Crossref PubMed Scopus (443) Google Scholar). The crystal structure of SMase I (sphingomyelin phosphodiesterase; E.C. 3.1.4.12), one of the sphingomyelinase D isoforms from L. laeta venom (determined at 1.75 Å), provides a structural basis for understanding the role of the metal ion binding and the acid-base catalytic mechanism.EXPERIMENTAL PROCEDURESSphingomyelinase Expression—L. laeta SMase I (GenBank™ accession number AY093599) was expressed in Escherichia coli strain BL21 (DE3) as a fusion protein composed of the mature SMase with an N-terminal extension containing a His6 tag (15.Fernandes-Pedrosa M.F. Junqueira de Azevedo I.L. Gonçalves-de-Andrade R.M. van den Berg C.W. Ramos C.R. Ho P.L. Tambourgi D.V. Biochem. Biophys. Res. Commun. 2002; 298: 638-645Crossref PubMed Scopus (96) Google Scholar). Recombinant SMase I was purified from the soluble fraction of cell lysates on a Ni(II)-chelating-Sepharose Fast Flow column (Amersham Biosciences). Recombinant protein was eluted (elution buffer: 100 mm Tris-HCl, pH 8.0, 300 mm NaCl, 0.8 m imidazole) at >95% purity and dialyzed against phosphate-buffered saline, pH 7.2 (10 mm sodium phosphate, 150 mm NaCl). Dynamic light-scattering experiments carried out in the above buffer at 293 K (DynaPro 801-Protein Solutions) indicated that the protein was monomeric in solution.Crystallization, Heavy Atom Derivative, and Data Collection—Initial crystals of L. laeta SMase I were obtained by the hanging drop vapor diffusion method in which 2-μl drops containing 1 μl of the protein solution (5 mg ml–1 in 25 mm Hepes, pH 7.5) were equilibrated against a reservoir solution containing 8 mm Hepes and 0.9 m trisodium citrate (pH 7.5) (31.Zela S.P. Fernandes-Pedrosa M.F. Murakami M.T. De Andrade S.A. Arni R.K. Tambourgi D.V. Acta Crystallogr. Sect. D Biol. Crystallogr. 2004; 60: 1112-1114Crossref PubMed Scopus (5) Google Scholar). Subsequently, crystals obtained from 2.7 m ammonium sulfate (pH 5.6) were easier to reproduce and were used for structure determination. Cryo-conditions included the addition of 20% (w/v) glycerol to the reservoir solution. The native crystals belong to the space group P65 with cell parameters of a = b = 139.82 Å and c = 113.46 Å, and the asymmetric unit contains four molecules with a solvent content of 52% (VM = 2.6 Å3 Da–1). The iodine derivative for the "quick cryo-soaking" method was prepared by soaking a single crystal in the cryo-solution, which additionally contained 0.5 m iodine chloride, for 20 min. Diffraction intensities for the native crystal were measured by a MARCCD detector at the protein crystallography beam line at the Brazilian National Synchrotron Light Source (LNLS, Campinas, Brazil), and the derivative diffraction data were collected using x-rays generated by a Rigaku RU300 rotating anode source equipped with Osmic confocal mirrors. Diffraction intensities were measured using a MAR 345 imaging plate detector. Data were scaled and reduced using DENZO/SCALEPACK (32.Otwinowski Z. Minor W. Methods Enzymol. 1997; 276: 307-326Crossref Scopus (38361) Google Scholar); the data collection and processing statistics are presented in Table I.Table IData collection and refinement statistics of SMase INative-SMase IIodine-SMase ICrystal preparation Cryprotectant solutionMother liquor + 20% glycerolMother liquor + 20% glycerol + 0.5 m NaI Soaking time60 s20 minData collection Wavelength (Å)1.431.54 Space groupP65P65 Unit cell parameters (Å)a = b = 139.82, c = 113.46a = b = 142.59, c = 115.36 Resolution (Å)30.0-1.75 (1.79-1.75)30.0-2.10 (2.15-2.10) No. of molecules in arbitrary units4 Solvent content (%)52 Vm (Å3Da−1)2.6 No. of reflections2,578,4171,580,276 No. of unique reflectionsaMultiplicities of the derivative data sets were calculated with the Friedel-related reflections treated separately. Multiplicity of the native data set was calculated with the Friedel pairs treated as equivalent.125,875151,663 I/σ(I)22.0 (3.4)cStatistical values for the highest resolution shells are given in parentheses.11.3 (2.4)cStatistical values for the highest resolution shells are given in parentheses. Multiplicity20.5 (6.5)10.4 (3.5) Completeness (%)99.5 (99.5)99.0 (98.2) Rmerge bRmerge = Σ|I(h)I - {I(h)}|/Σ{I(h)}, where Ih is the observed intensity of the ith measurement of reflection h and {I(h)} is the mean intensity of reflection h calculated after scaling. (%)6.4 (43.9)10.8 (48.3)Structure refinement statistics Rfactor (%)18.6 Rfree (%)22.5 Root mean square deviation bond distances (Å)0.013 Root mean square deviation bond angles (°)1.549 Average B-factors (Å2)24.2a Multiplicities of the derivative data sets were calculated with the Friedel-related reflections treated separately. Multiplicity of the native data set was calculated with the Friedel pairs treated as equivalent.b Rmerge = Σ|I(h)I - {I(h)}|/Σ{I(h)}, where Ih is the observed intensity of the ith measurement of reflection h and {I(h)} is the mean intensity of reflection h calculated after scaling.c Statistical values for the highest resolution shells are given in parentheses. Open table in a new tab Structure Determination and Refinement—The quick cryo-soaking method (33.Dauter Z. Dauter M. Rajashankar K.R. Acta Crystallogr. Sect. D Biol. Crystallogr. 2000; 56: 232-237Crossref PubMed Scopus (281) Google Scholar, 34.Nagem R.A. Dauter Z. Polikarpov I. Acta Crystallogr. Sect. D Biol. Crystallogr. 2001; 57: 996-1002Crossref PubMed Scopus (65) Google Scholar) was used for derivatization and phasing. The structure was determined at 1.95 Å using the single isomorphous replacement anomalous dispersion method, and the anomalous differences were used to locate 41 iodine sites with the SHELXD program (35.Schneider T.R. Sheldrick G.M. Acta Crystallogr. Sect. D Biol. Crystallogr. 2002; 58: 1772-1779Crossref PubMed Scopus (1574) Google Scholar) by integrated-direct and Patterson methods. These heavy atom positions were used without further refinement to estimate phases that were subsequently extended to 1.75-Å resolution applying the sphere of influence algorithm as incorporated in the SHELXE program (35.Schneider T.R. Sheldrick G.M. Acta Crystallogr. Sect. D Biol. Crystallogr. 2002; 58: 1772-1779Crossref PubMed Scopus (1574) Google Scholar), and the electron density was improved by solvent flattening with SOLOMON (36.Abrahams J.P. Leslie A.J.W. Acta Crystallogr. Sect. D. 1996; 52: 30-42Crossref PubMed Scopus (1140) Google Scholar). The deduced amino acid sequence based on the L. laeta SMase I gene (GenBank™ accession number AAM21154) was utilized for automatic model building into the 1.75-Å resolution electron density map with the ARP/wARP program (37.Perrakis A. Morris R.J.H. Lamzin V. Nat. Struct. Biol. 1999; 6: 458-463Crossref PubMed Scopus (2562) Google Scholar), which was able to trace 97% of the molecule. The refinement was initiated at a 2.0-Å resolution with an R-factor of 22.2% (Rfree = 26.4%). Initial cycles of refinement involved translation, libration, and screw rotation, a restrained and overall B-factor refinement that was carried out by REFMAC5 (38.Murshudov G.N. Vagin A.A. Dodson E.J. Acta Crystallogr. Sect. D. 1997; 53: 240-255Crossref PubMed Scopus (13776) Google Scholar) with the inclusion of non-crystallographic restraints. After each cycle of refinement, the model was inspected and manually adjusted to correspond to the computed σA-weighted (2Fo – Fc)- and (Fo – Fc)-type electron density maps using the program TURBO FRODO (Biographics, Marseille, France). In the later cycles, the non-crystallographic restraints were relaxed, and individual isotropic B-factors were refined. Solvent water molecules were added manually at the position of positive peaks (>3σ) in the difference Fourier maps, taking into consideration hydrogen bonding potential. In the final cycles of refinement, additional density was observed in the difference electron density maps, which was attributed to the presence of Mg2+, sulfate ions, and Hepes molecules.RESULTSThe refinement of the structure of SMase I from L. laeta converged to a crystallographic residual of 18.6% (Rfree = 22.5% for 5% of the data) for all data between 30.0 and 1.75 Å (no σ or intensity cutoff; 99.5% data completeness). The residual electron density that was observed was attributed to the presence of a Mg2+ ion based on the temperature factor (B-factor = 7.5 Å2) and coordination (Figs. 1 and 2A). Because the concentration of the phosphate in the dialysis buffer (10 mm) was lower than the sulfate concentration (2.6 m) used in crystallization, the tetrahedrally shaped residual density observed in close proximity to the Mg2+ binding site was considered to represent an SO4– ion (Fig. 2A). Although hydrolytic activity is Mg2+-dependent and this site is surrounded by highly conserved amino acids, it is considered to represent the active site, and the SO4– ion likely mimics the binding of the phosphate group of the substrate.Fig. 2A, stereo view of the amino acids and hydrogen bonding in the catalytic and metal ion binding (green sphere) sites; the electron density (blue) in the 2Fo – Fc map is contoured at 2.0σ. B, schematic representation of the principal hydrogen bonds to the sulfate and metal ion.View Large Image Figure ViewerDownload Hi-res image Download (PPT)The refined model of SMase I contains 1140 amino acid residues, 4 Mg2+ ions, 23 sulfate ions, 3 Hepes, and 1018 solvent water molecules. An analysis of the stereochemistry (PROCHECK) (39.Laskowski R.A. MacArthur M.W. Moss D.S. Thornton J.M. J. Appl. Crystallogr. 1993; 26: 283-291Crossref Google Scholar) of the final model indicates that the main-chain dihedral angles for all residues are located in the permitted regions of the Ramachandran diagram and that the root mean square deviations from ideal values are distributed within the expected ranges for a well refined structure (Table I).The fundamental structural unit of SMase I is formed by a distorted triose phosphate isomerase or (α/β)8 barrel (surface area = 11,254 Å2) with the insertion of additional β-strands and α-helices (Figs. 1 and 3). The N and C termini flank one side of the barrel, and the N terminus leads directly to the first β-strand, whereas the C terminus contains a short helix (8′) and a β-strand (H′) and caps the torus of the barrel (Figs. 1 and 3). The asymmetric unit contains four molecules and can be considered to be made up of two dimers. The monomers forming the dimer are related by a 2-fold axis of rotation perpendicular to the barrel (Fig. 4) and results in 3402 Å2 (or 33%) of the surface area of each monomer being buried. Dimerization is likely an artifact of the crystal packing interactions, because the dynamic light-scattering experiments indicate that the protein is monomeric in solution.Fig. 3Topology schematic of L. laeta SMase I. The β-strands (arrows) and α-helices (cylinders) forming the (α/β)8 barrel are labeled A–H and 1–8 respectively. The β-strands and α-helices not belonging to the core are primed. The positions of the catalytic loop B (blue), variable loop E (green), flexible loop F (red), and the disulfide bridge (S–S) are indicated. The approximate relative positions of the amino acids involved in catalysis and Mg2+ ion binding are indicated.View Large Image Figure ViewerDownload Hi-res image Download (PPT)Fig. 4Ribbon representation of the dimer. The amino acids His12, Glu32, Asp34, His47, and Asp91 are represented by balls and sticks, and the Mg2+ ion is represented as a green sphere. Residues in the catalytic (loop B), variable (loop E), and the flexible (loop F) loops are blue, green, and red, respectively. The N and C termini are labeled and are brown.View Large Image Figure ViewerDownload Hi-res image Download (PPT)The opposite face of the barrel is surrounded by a ring of negatively charged amino acids and hydrophobic loops (Fig. 1). The active site pocket contains His12, Glu32, Asp34, Asp91, His47, Asp52, Trp230, Asp233, and Asn252, which are fully conserved in the Loxosceles species SMases D isoforms (Fig. 5). Mutagenesis studies of Mg2+-dependent neutral SMase (40.Obama T. Fujii S. Ikezawa H. Ikeda K. Imagawa M. Tsukamoto K. Biol. Pharm. Bull. 2003; 26: 920-926Crossref PubMed Scopus (15) Google Scholar) and the crystal structure of phospholipase D (41.Stuckey J.A. Dixon J.E. Nat. Struct. Biol. 1999; 6: 278-284Crossref PubMed Scopus (183) Google Scholar) indicate the involvement of two histidine residues that are in close proximity to the metal ion binding site in the acid-base catalytic mechanism. Based on the structural results, His12 and His47 of SMase D have been identified as the key residues for catalysis and are assisted by a hydrogen bond network that involves Asp52, Asn252, and Asp233. The metal ion is coordinated by Glu32, Asp34, Asp91, and solvent molecules.Fig. 5Structure-based multiple sequence alignments of the SMases D isoforms performed using three-dimensional Coffee 2004 ( 49.O′Sullivan O. Suhre K. Abergel C. Higgins D.G. Notredam C. J. Mol. Biol. 2004; 340: 385-395Crossref PubMed Scopus (255) Google Scholar). L. laeta: SMase I, H10, and H13 (GenBank™ accession numbers AY093599, AY093600 and AY093601, respectively) (15.Fernandes-Pedrosa M.F. Junqueira de Azevedo I.L. Gonçalves-de-Andrade R.M. van den Berg C.W. Ramos C.R. Ho P.L. Tambourgi D.V. Biochem. Biophys. Res. Commun. 2002; 298: 638-645Crossref PubMed Scopus (96) Google Scholar); L. intermedia: P1 and P2 (accession numbers AY304471 and AY304472, respectively) (14.Tambourgi D.V. Fernandes-Pedrosa M.F. van den Berg C.W. Gonçalves-de-Andrade R.M. Ferracini M. Paixão-Cavalcante D. Morgan B.P. Rushmere N.K. Mol. Immunol. 2004; 41 (F.): 831-840MCrossref PubMed Scopus (61) Google Scholar); L. reclusa: Lr1 and Lr2 (accession numbers AY559846 and AY559847, respectively) (19.Soucek A. Michalec C. Souckova A. Biochim. Biophys. Acta. 1967; 144: 180-182Crossref PubMed Scopus (28) Google Scholar); Loxosceles boneti: Lb1 and Lb3 (accession numbers AY559844 and AY559845, respectively) (50.Ramos-Cerrillo B. Olivera A. Odell G.V. Zamudio F. Paniagua-Solis J. Alagon A. Stock R.P. Toxicon. 2004; 44: 507-514Crossref PubMed Scopus (61) Google Scholar) and C. pseudotuberculosis: PLD (accession number L16586) (21.McNamara P.J. Cuevas W.A. Songer J.G. Gene. 1995; 156: 113-118Crossref PubMed Scopus (81) Google Scholar). Residues involved in catalysis or metal ion coordination are shaded gray. Amino acids in loops B, E, and F are boxed. Sequence numbers indicated are for L. laeta SMase I.View Large Image Figure ViewerDownload Hi-res image Download (PPT)His12 is located at the tip of the first β-strand (strand A, Figs. 1 and 3), and Nϵ2 forms a hydrogen bond to O2 of the bound sulfate ion. His12Nδ1 is hydrogen bonded to Asn252Oδ1 and Asn252Nδ2 is further hydrogen bonded to Asp52Oδ2, and Asp233Oδ2 (Fig. 2).The catalytic loop (loop B, residues 46–60) is stabilized at the tip by a disulfide bridge (Cys51-Cys57, Figs. 3 and 6), which is a conserved feature of all spider SMases D (Fig. 5), and His47 is located at the base of the loop. Multiple hydrogen bonds are formed between Arg55NH1 and the main-chain carbonyl oxygen of His12 and Arg55NH2 and Met13O (Fig. 6), and additionally Arg55NH1 also bonds Asp252Oδ1. A structural sulfate ion binds via O1 to Arg55Nϵ and the main chain amide nitrogen of Asp56. O4 of the sulfate ion binds two solvent molecules, both of which are hydrogen bonded to Arg59NH1. Arg59Nϵ binds a molecule of Hepes, the latter interacting with the indole of Trp60 located at the base of the catalytic loop (loop B) on the external surface of the barrel. This network of bonds ensures the orientation of the catalytic loop in relation to the active site. His47Nϵ2 is hydrogen bonded to O1 and O3 of the sulfate ion located in the catalytic site, and His47Nδ1 interacts with the carbonyl oxygen atom of Gly48 (Fig. 2B).Fig. 6Network of hydrogen bonds (dashed red lines) participating in the stabilization of the catalytic loop (loop B). The structural SO4– ion, disulfide bridge (yellow bond), and solvent molecules (red spheres) are also included.View Large Image Figure ViewerDownload Hi-res image Download (PPT)The bound Mg2+ ion (Fig. 2) is octahedrally coordinated (mean Mg2+–O distance of 1.98 Å), equatorially by carboxylate oxygens from t
Referência(s)