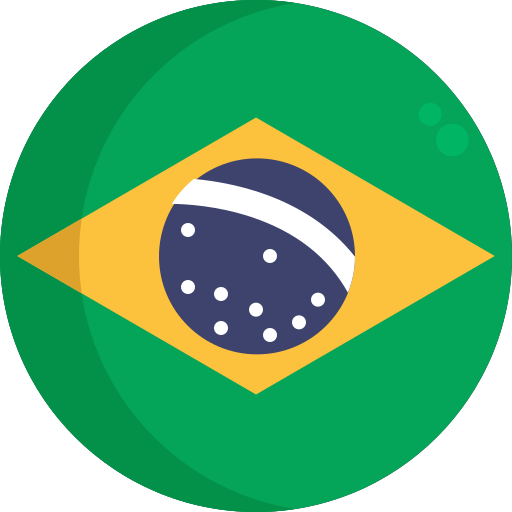
Unmasking a Growth-promoting Effect of the Adrenocorticotropic Hormone in Y1 Mouse Adrenocortical Tumor Cells
1997; Elsevier BV; Volume: 272; Issue: 47 Linguagem: Inglês
10.1074/jbc.272.47.29886
ISSN1083-351X
AutoresClaudimara Ferini Pacicco Lotfi, Zana Todorovic, Hugo A. Armelin, Bernard P. Schimmer,
Tópico(s)Adrenal and Paraganglionic Tumors
ResumoThe adrenocorticotropic hormone (ACTH) inhibits the growth of Y1 mouse adrenocortical tumor cells as well as normal adrenocortical cells in culture but stimulates adrenocortical cell growth in vivo. In this study, we investigated this paradoxical effect of ACTH on cell proliferation in Y1 adrenal cells and have unmasked a growth-promoting effect of the hormone. Y1 cells were arrested in the G1 phase of the cell cycle by serum starvation and monitored for progression through S phase by measuring [3H]thymidine incorporation into DNA and by measuring the number of nuclei labeled with bromodeoxyuridine. Y1 cells were stimulated to progress through S phase and to divide after a brief pulse of ACTH (up to 2 h). This effect of ACTH appeared to be cAMP independent, since ACTH also induced cell cycle progression in Kin-8, a Y1 mutant with defective cAMP-dependent protein kinase activity. The growth-promoting effect of ACTH in Y1 was preceded by the rapid activation of p44 and p42 mitogen-activated protein kinases and by the accumulation of c-FOS protein. In contrast, continuous treatment with ACTH (14 h) inhibited cell cycle progression in Y1 cells by a cAMP-dependent pathway. The inhibitory effect of ACTH mapped to the midpoint of G1. Together, the results demonstrate a dual effect of ACTH on cell cycle progress, a cAMP-independent growth-promoting effect early in G1possibly mediated by mitogen-activated protein kinase and c-FOS, and a cAMP-dependent inhibitory effect at mid-G1. It is suggested that the growth-inhibitory effect of ACTH at mid-G1 represents an ACTH-regulated check point that limits cell cycle progression. The adrenocorticotropic hormone (ACTH) inhibits the growth of Y1 mouse adrenocortical tumor cells as well as normal adrenocortical cells in culture but stimulates adrenocortical cell growth in vivo. In this study, we investigated this paradoxical effect of ACTH on cell proliferation in Y1 adrenal cells and have unmasked a growth-promoting effect of the hormone. Y1 cells were arrested in the G1 phase of the cell cycle by serum starvation and monitored for progression through S phase by measuring [3H]thymidine incorporation into DNA and by measuring the number of nuclei labeled with bromodeoxyuridine. Y1 cells were stimulated to progress through S phase and to divide after a brief pulse of ACTH (up to 2 h). This effect of ACTH appeared to be cAMP independent, since ACTH also induced cell cycle progression in Kin-8, a Y1 mutant with defective cAMP-dependent protein kinase activity. The growth-promoting effect of ACTH in Y1 was preceded by the rapid activation of p44 and p42 mitogen-activated protein kinases and by the accumulation of c-FOS protein. In contrast, continuous treatment with ACTH (14 h) inhibited cell cycle progression in Y1 cells by a cAMP-dependent pathway. The inhibitory effect of ACTH mapped to the midpoint of G1. Together, the results demonstrate a dual effect of ACTH on cell cycle progress, a cAMP-independent growth-promoting effect early in G1possibly mediated by mitogen-activated protein kinase and c-FOS, and a cAMP-dependent inhibitory effect at mid-G1. It is suggested that the growth-inhibitory effect of ACTH at mid-G1 represents an ACTH-regulated check point that limits cell cycle progression. The growth-inhibitory effects of the adrenocorticotropic hormone (ACTH) 1The abbreviations used are: ACTH, adrenocorticotropic hormone; 8-Br-cAMP, 8-bromo-cAMP; DMEM, Dulbecco's modified Eagle's Medium; Erk1, p44 MAP kinase; Erk2, p42 MAP kinase; FGF, fibroblast growth factor; G protein, guanyl nucleotide-binding regulatory G protein; MAP kinase, mitogen-activated protein kinase; PKA, cAMPdependent protein kinase; PMA, phorbol 12-myristate 13-acetate; BrdUrd, bromodeoxyuridine. on adrenal cellsin vitro are well documented. ACTH-induced inhibition of cell proliferation has been observed in the Y1 mouse adrenocortical tumor cell line (1Masui H. Garren L.D. Proc. Natl. Acad. Sci. U. S. A. 1971; 68: 3206-3210Crossref PubMed Scopus (140) Google Scholar) as well as in normal adrenocortical cells isolated from a variety of species including rat, cow, and human (for review, see Ref. 2Hornsby P.J. Endocr. Res. 1985; 10: 259-281Crossref Scopus (49) Google Scholar). ACTH arrests dividing adrenal cells by interfering with progression through the G1 phase of the cell cycle (3Kimura E. Armelin M.C.S. Armelin H.A. Rev. Bras. Genet. 1983; 2: 211-227Google Scholar) and inhibits the initiation of DNA synthesis in G1-arrested cells following addition of serum or growth factors (4Weidman R.E. Gill G.N. J. Cell. Physiol. 1977; 90: 91-103Crossref PubMed Scopus (47) Google Scholar, 5Armelin M.C.S. Gambarini A.G. Armelin H.A. J. Cell. Physiol. 1977; 93: 1-9Crossref PubMed Scopus (24) Google Scholar). Several lines of evidence indicate that the growth-inhibitory effect of ACTH is mediated by cAMP with the most compelling data arising from studies of Y1 adrenal tumor cells harboring dominant inhibitory mutations in cAMP-dependent protein kinase (PKA) that specifically disrupt cAMP-dependent signaling pathways (6Olson M.F. Krolczyk A.J. Gorman K.B. Steinberg R.A. Schimmer B.P. Mol. Endocrinol. 1993; 7: 477-487Crossref PubMed Scopus (0) Google Scholar). These PKA mutants are resistant to the growth-inhibitory actions of ACTH and cAMP analogs (7Schimmer B.P. Schulz P. Endocr. Res. 1985; 11: 199-209Crossref PubMed Scopus (24) Google Scholar, 8Armelin H.A. Lotfi C.F.P. Lepique A.P. Endocr. Res. 1996; 22: 373-383Crossref PubMed Scopus (19) Google Scholar), indicating that cAMP and PKA are obligatory components of this effect of ACTH on cell proliferation. The inhibition of proliferation seen in isolated adrenocortical cells contrasts sharply with the growth-promoting effects of ACTH on the adrenal gland in vivo and has led to the widely held view that ACTH serves as an indirect mitogen for the adrenal cortex in intact animals (2Hornsby P.J. Endocr. Res. 1985; 10: 259-281Crossref Scopus (49) Google Scholar). Paradoxically, however, ACTH induces expression of genes often associated with enhanced cell proliferation such as ornithine decarboxylase (9Kudlow J.E. Rae P.A. Gutmann N.S. Schimmer B.P. Burrow G.N. Proc. Natl. Acad. Sci. U. S. A. 1980; 77: 2767-2771Crossref PubMed Scopus (32) Google Scholar) and fos and jun protooncogenes (10Kimura E. Armelin H.A. J. Biol. Chem. 1990; 265: 3518-3521Abstract Full Text PDF PubMed Google Scholar, 11Kimura E. Sonobe M.H. Armelin M.C.S. Armelin H.A. Mol. Endocrinol. 1993; 7: 1463-1471Crossref PubMed Scopus (0) Google Scholar, 12Viard I. Penhoat A. Ouali R. Langlois D. Begeot M. Saez J.M. J. Steroid Biochem. Mol. Biol. 1994; 50: 219-224Crossref PubMed Scopus (12) Google Scholar) in isolated adrenocortical cells, raising the possibility of an underlying growth-promoting action of the hormone. The MAP kinase cascade, an important regulator of cell cycle progression, has been used recently as a biochemical marker to evaluate the status of hormones and growth factors as mitogens. Activation of the MAP kinase pathway is involved in the mitogenic effects of growth factors such as epidermal growth factor, platelet-derived growth factor, and FGF (13L'Allemain G. Pouyssegur J. Weber M.J. Cell Regul. 1991; 2: 675-684Crossref PubMed Scopus (92) Google Scholar, 14Malarkey K. Belham C.M. Paul A. Graham A. McLees A. Scott P.H. Plevin R. Biochem. J. 1995; 309: 361-375Crossref PubMed Scopus (266) Google Scholar), acting via receptor tyrosine kinases and also appears to mediate the mitogenic effects of thyrotropin on thyrocytes (15Saunier B. Tournier C. Jacquemin C. Pierre M. J. Biol. Chem. 1995; 270: 3693-3697Abstract Full Text Full Text PDF PubMed Scopus (40) Google Scholar), angiotensin II on smooth muscle cells (16Duff J.L. Berk B.C. Corson M.A. Biochem. Biophys. Res. Commun. 1992; 188: 257-264Crossref PubMed Scopus (197) Google Scholar), and thrombin on fibroblasts (17Trejo J. Connolly A.J. Coughlin S.R. J. Biol. Chem. 1996; 271: 21536-21541Abstract Full Text Full Text PDF PubMed Scopus (114) Google Scholar), each acting through a G protein-coupled receptor. Conversely, inhibition of the MAP kinase cascade accompanies the growth-inhibitory effects of cAMP observed in fibroblasts and other cell types (for review, see Ref. 18Campbell J.S. Seger R. Graves J.D. Graves L.M. Jensen A.M. Krebs E.G. Recent Prog. Horm. Res. 1995; 50: 131-159PubMed Google Scholar). In the present study, we examined the regulation of the MAP kinase pathway in Y1 mouse adrenocortical tumor cells to reconcile the growth-inhibiting effect of ACTH in vitro with the conflicting biochemical data that suggests an underlying mitogenic effect of the hormone. Although we expected that ACTH would inhibit MAP kinase activity in Y1 cells, consistent with the growth-inhibitory effects of the hormone, we find that ACTH activates the MAP kinase cascade via a signaling mechanism that is cAMP-independent. This effect of ACTH on MAP kinase prompted us to reexamine the effects of ACTH on Y1 cell proliferation. We find that ACTH promotes the transition of Y1 cells from G1 to S in a cAMP-independent manner and stimulates cell division when administered to Y1 cells as a short pulse early in the G1 phase of the cell cycle. In contrast, administration of ACTH later in the cell cycle inhibits the transition of cells from G1 to S. These results suggest that we have unmasked a cAMP-independent, growth-promoting effect of ACTH that has new implications for the mitogenic effects of the hormone on the adrenal cortex. The cells used in this study are the Y1 mouse adrenocortical tumor cell line (19Yasumura Y. Buonassisi V. Sato G. Cancer Res. 1966; 26: 529-535PubMed Google Scholar) and a cAMP-resistant, PKA-defective mutant of the Y1 cell line designated Kin-8 (20Rae P.A. Gutmann N.S. Tsao J. Schimmer B.P. Proc. Natl. Acad. Sci. U. S. A. 1979; 76: 1896-1900Crossref PubMed Scopus (129) Google Scholar). Cells routinely were grown either in Ham's nutrient mixture F10 supplemented with 15% heat-inactivated horse serum and 2.5% heat-inactivated fetal bovine serum or in DMEM with 10% fetal bovine serum as described in detail previously (21Schimmer B.P. Methods Enzymol. 1985; 109: 350-356Crossref PubMed Scopus (29) Google Scholar). Cells were arrested early in the G1phase of the cell cycle by incubation in serum-deficient medium for the times specified. All cultures were maintained at 36.5 °C in a humidified atmosphere of 5% CO2, 95% air. Cells were replicate plated at a density of 5 × 105 cells per 10-cm tissue culture dish, cultured for 4 days in F10 growth medium plus serum, and then transferred to serum-free alpha minimal essential medium for 72 h to induce growth arrest. Cells then were treated with various reagents to modulate the MAP kinase cascade, rinsed twice with phosphate-buffered saline, and solubilized in 0.5 ml of RIPA buffer containing 50 mm Tris-HCl (pH 8.0), 150 mmNaCl, 5 mm EDTA, 1% (v/v) Nonidet P-40, 0.5% (w/v) sodium deoxycholate, 0.1% SDS, protease inhibitors (0.1 mmphenylmethanesulfonyl fluoride, 10 μg/ml leupeptin, 10 μg/ml soybean trypsin inhibitor, 10 μg/ml benzamidine, 5 μg/ml aprotinin), and phosphatase inhibitors (10 mm sodium fluoride, 10 mm disodium pyrophosphate, and 1 mm sodium orthovanadate) (22Koch W.J. Hawes B.E. Allen L.F. Lefkowitz R.J. Proc. Natl. Acad. Sci. U. S. A. 1994; 91: 12706-12710Crossref PubMed Scopus (409) Google Scholar). Samples were clarified by centrifugation at 4 °C. Samples (10 μg of protein) were boiled in SDS sample buffer for 3–5 min, electrophoresed on 10% polyacrylamide gels in the presence of SDS (23Laemmli U.K. Nature. 1970; 227: 680-685Crossref PubMed Scopus (207537) Google Scholar), and electroblotted onto polyvinylidene difluoride membranes (NEN Life Science Products) using a Bio-Rad transblot apparatus. Phosphorylated forms of MAP kinase were detected by immunoblotting using a PhosphoPlus MAPK antibody kit (New England Biolabs, Missisauga, Ontario). The kit provides a primary rabbit antibody that specifically recognizes the tyrosine-phosphorylated forms of p44 MAP kinase (Erk1) and p42 MAP kinase (Erk2) and a secondary alkaline phosphatase-conjugated anti-rabbit antibody for chemiluminescent detection. The incorporation of [methyl-3H]thymidine into DNA was determined essentially as described previously (5Armelin M.C.S. Gambarini A.G. Armelin H.A. J. Cell. Physiol. 1977; 93: 1-9Crossref PubMed Scopus (24) Google Scholar, 24Gill G.N. Weidman E.R. J. Cell. Physiol. 1977; 92: 65-75Crossref PubMed Scopus (18) Google Scholar). Cells were replicate plated at a density of 8 × 104 cells per 6-cm tissue culture dish and cultured for 2 days in growth medium plus serum to ensure logarithmic growth. Cells were transferred to alpha minimal essential medium without serum for 72 h to arrest cells early in the G1 phase of the cell cycle. Cells then were rinsed and incubated for another 14 h in 2 ml of fresh alpha minimal essential medium with various additions as indicated. During the last 2 h of incubation, cells were pulsed with 2 μCi/ml of [methyl-3H]thymidine (NEN Life Science Products; 20 Ci/mmol) and collected on glass fiber discs by filtration. DNA was precipitated on the filters using 5% trichloroacetic acid; samples were washed with 95% ethanol, dried, and counted by liquid scintillation spectrometry. Approximately 2 × 104 cells were seeded on coverslips in DMEM with serum and then serum-starved for 48 h. Cells were treated with various reagents to promote transition of cells from G1 to the S phase of the cell cycle and then incubated with 100 μmBrdUrd to label S phase nuclei as described in the appropriate figure legends. Cells were fixed with cold methanol for 10 min and stored. BrdUrd-labeled nuclei were estimated by indirect immunofluorescence. Coverslips with fixed cells were incubated in 1.5 n HCl for 30 min, inverted onto a 40-μl drop of mouse anti-BrdUrd antibody (Sigma), and incubated for 30 min at room temperature. Coverslips were washed three times in phosphate-buffered saline and immune complexes were detected with a fluorescein isothiocyanate-conjugated mouse IgG secondary antibody (Sigma) using the same procedure. Next, the coverslips were incubated with 4′,6-diamidino-2-phenylindole (5 μg/ml) for 20 min to fluorescently stain total nuclei and then examined under a Nikon Fluophot microscope using two excitation filters: UV 330–380 to visualize 4′,6-diamidino-2-phenylindole-stained total nuclei and IF420–490 to visualize the BrdUrd-labeled nuclei stained with fluorescein isothiocyanate. The coverslips were randomly coded, and the fluorescent nuclei were counted blindly. Between 500 and 750 nuclei were counted per coverslip. Regulation of the MAP kinase pathway in Y1 cells was monitored by measuring the phosphorylation and activation of two MAP kinase isoforms, Erk1 and Erk2. Y1 cells were maintained in serum-free medium for 72 h to arrest cell growth early in G1. Cells were then transferred to medium that was fully supplemented with serum, harvested at different time periods, and subjected to Western blot analysis using an antibody that specifically recognizes tyrosine-phosphorylated forms of Erk1 and Erk2. As shown in Fig.1 a, serum rapidly and transiently stimulated the appearance of phosphorylated forms of Erk1 and Erk2. These phosphorylated forms of MAP kinase were detectable within 1 min after serum addition, reached a maximum after 5 min, and declined toward control levels by 20 min. FGF, another mitogen for Y1 cells (25Gospodarowicz D. Handley H.H. Endocrinology. 1975; 97: 102-107Crossref PubMed Scopus (61) Google Scholar), also stimulated the phosphorylation of Erk1 and Erk2 but with somewhat delayed kinetics (Fig. 1 b). The appearance of phosphorylated forms of MAP kinase in response to serum or FGF reflected activation of MAP kinase as determined by direct measures of enzymatic activity (data not shown). To determine if the growth-inhibitory effects of ACTH and 8-Br-cAMP in Y1 cells were associated with inhibition of the MAP kinase cascade, Y1 cells were preincubated with porcine ACTH (Acthar; Rhône-Poulenc Rorer Canada Inc., Montreal, Quebec) or 8-Br-cAMP for 10 min and then treated with serum for varying periods of time. Although this same experimental paradigm had been used previously to correlate the growth-inhibitory effects of cAMP with an inhibitory effect on the MAP kinase pathway in rat and hamster fibroblasts (26Cook S.J. McCormick F. Science. 1993; 262: 1069-1072Crossref PubMed Scopus (865) Google Scholar, 27McKenzie F.R. Pouyssegur J. J. Biol. Chem. 1996; 271: 13476-13483Abstract Full Text Full Text PDF PubMed Scopus (90) Google Scholar), pretreatment of Y1 adrenal cells with ACTH or 8-Br-cAMP failed to inhibit serum-stimulated MAP kinase phosphorylation (Fig. 1, c andd). To the contrary, the addition of ACTH to Y1 cells arrested by serum starvation rapidly stimulated the phosphorylation of Erk1 and Erk2 (Fig. 2 a) and increased MAP kinase activity (data not shown). The hormone was effective at concentrations as low as 0.1 microunits/ml. Synthetic human ACTH1–39 also stimulated MAP kinase phosphorylation (Fig. 2 a) over a similar concentration range (data not shown), excluding the possibility that the observed effects were due to growth factors contaminating the pituitary-derived Acthar preparation. The concentrations of ACTH that effectively stimulated MAP kinase activity were 50-fold lower than the concentrations of hormone required to produce detectable changes in cAMP levels in these cells (28Schimmer B.P. Zimmerman A.E. Mol. Cell. Endocrinol. 1976; 4: 263-270Crossref PubMed Scopus (29) Google Scholar), raising the possibility that ACTH exerted its effects on MAP kinase via a cAMP-independent pathway. To test this hypothesis, we examined the ability of ACTH to stimulate MAP kinase phosphorylation in the cAMP-resistant, PKA-defective mutant Kin-8. As shown in Fig.2 b, ACTH effectively activated MAP kinase phosphorylation in the Kin-8 mutant over the same concentration range as seen in parent Y1 cells, supporting the hypothesis that activation of the MAP kinase cascade occurred through a cAMP-independent mechanism. In both parental Y1 cells and the PKA-defective mutant, Kin-8, the effect of ACTH was mimicked by the phorbol ester PMA (Sigma) but not by the inactive 4α-hydroxyl isomer of PMA, implicating a protein kinase C-dependent pathway of regulation (Fig. 2, c andd). Although MAP kinase phosphorylation also was stimulated by 8-Br-cAMP, a similar effect was achieved with 5′-AMP (Fig. 2,c and d), suggesting that the action was not specific for the cyclic nucleotide and possibly involved signaling via purinergic receptors. The ability of serum-starved Y1 cells to progress from the G1 phase of the cell cycle to S phase was monitored by measuring [3H]thymidine incorporation into DNA or by measuring the percentage of nuclei labeled with BrdUrd. Incubation of Y1 cells with serum for 14 h led to a 15-fold increase in [3H]thymidine incorporation into DNA (TableI). As determined from BrdUrd-labeling experiments, the changes in [3H]thymidine incorporation into Y1 cell DNA reflected the progression of cells from the G1 phase of the cell cycle to S phase. As shown in Fig.3, fetal bovine serum and FGF both stimulated the entry of Y1 cells into S phase with a lag of approximately 8 h and resulted in the labeling of approximately 80% of nuclei after 24 h. ACTH, 8-Br-cAMP, or PMA, when added together with serum during the entire incubation period, dramatically and significantly reduced the amount of [3H]thymidine incorporated (Table I). Even when added alone for 14 h, ACTH caused a significant reduction in [3H]thymidine incorporation compared with growth-arrested Y1 cells.Table IProlonged incubation of Y1 cells with ACTH, 8-Br-cAMP or PMA inhibits incorporation of [3H]thymidine into DNATreatmentThymidine incorporationStatistical significanceNone1.0Serum15.0 ± 3.25p = 0.0081-ap values for significance relative to serum-starved controls.ACTH0.2 ± 0.04p < 0.0011-ap values for significance relative to serum-starved controls.ACTH + serum2.6 ± 0.42p < 0.0011-bp values for significance relative to serum-treated samples.8-Br-cAMP + serum0.20 ± 0.01p= 0.00011-bp values for significance relative to serum-treated samples.PMA + serum6.1 ± 1.9p < 0.0011-bp values for significance relative to serum-treated samples.Y1 cells in the logarithmic phase of growth (approximately 2 × 105 cells per 60-mm dish) were arrested in early G1 by serum starvation for 72 h. Cells then were rinsed and incubated in fresh serum-free medium or in medium supplemented with 15% horse serum and 2.5% fetal bovine serum for 14 h. During the last 2 h of incubation, cells were pulsed with [3H]thymidine (2 μCi/ml). Where indicated, ACTH (Acthar; 25 milliunits/ml), 8-Br-cAMP (3.0 mm) or PMA (0.1 μm) were included at the start of the incubation period. Values for thymidine incorporation represent fold changes ± S.E. relative to the serum-starved controls. Statistical significance was determined using Peritz'sF test, a robust multiple comparison test for statistical analysis of all differences among group means (41Harper J. Comput. Biol. Med. 1984; 14: 437-445Crossref PubMed Scopus (288) Google Scholar).1-a p values for significance relative to serum-starved controls.1-b p values for significance relative to serum-treated samples. Open table in a new tab Y1 cells in the logarithmic phase of growth (approximately 2 × 105 cells per 60-mm dish) were arrested in early G1 by serum starvation for 72 h. Cells then were rinsed and incubated in fresh serum-free medium or in medium supplemented with 15% horse serum and 2.5% fetal bovine serum for 14 h. During the last 2 h of incubation, cells were pulsed with [3H]thymidine (2 μCi/ml). Where indicated, ACTH (Acthar; 25 milliunits/ml), 8-Br-cAMP (3.0 mm) or PMA (0.1 μm) were included at the start of the incubation period. Values for thymidine incorporation represent fold changes ± S.E. relative to the serum-starved controls. Statistical significance was determined using Peritz'sF test, a robust multiple comparison test for statistical analysis of all differences among group means (41Harper J. Comput. Biol. Med. 1984; 14: 437-445Crossref PubMed Scopus (288) Google Scholar). The observation that ACTH rapidly activated the MAP kinase cascade when added to growth-arrested Y1 cells (Fig. 2) prompted us to reexamine the effects of ACTH on the cell cycle particularly when administered over a short period to coincide with the activation of MAP kinase. As shown in Fig.4, treatment of serum-starved Y1 cells with fetal bovine serum or FGF for varying periods of time caused a progressive increase in the percentage of nuclei labeled with BrdUrd, reaching a maximum stimulation of 3.5–5.5-fold (p < 0.05) after 24 h. At the end of this period, 80–90% of nuclei were labeled with BrdUrd. Using this experimental paradigm, we found that treatment with ACTH for short periods up to 2 h increased the labeling index of Y1 cells almost 2-fold (p < 0.05) and approached the effects of serum and FGF over the same periods (Fig.4). In contrast, treatments with ACTH for longer periods of time were inhibitory. A short pulse of ACTH also stimulated progression of Y1 cells from G1 to S as measured by incorporation of [3H]thymidine into DNA (TableII). Treatment of serum-starved Y1 cells with ACTH for 5 min produced a 4-fold increase in thymidine incorporation over controls, which approached the effects obtained with FGF over the same interval. Similar results were obtained after a brief exposure of Y1 cells to synthetic ACTH1–39 in equimolar amounts (data not shown), ensuring that the transition of cells from G1 to S resulted specifically from the hormone and not from another contaminating growth factor in the pituitary-derived hormone preparation.Table IITreatment of Y1 or Kin-8 cells with short pulses of ACTH or PMA stimulates incorporation of [3H]thymidine into DNACell lineTreatmentThymidine incorporationStatistical significanceY1None1.0Serum6.2 ± 0.45p = 0.0004FGF4.1 ± 0.30p = 0.007ACTH4.0 ± 0.95p = 0.001PMA2.1 ± 0.14p = 0.01Kin-8None1.0Serum5.0 ± 0.5p < 0.001FGF4.4 ± 0.35p < 0.001ACTH3.4 ± 0.5p = 0.005PMA4.3 ± 0.52p < 0.0006Cells were growth-arrested as described in Table I, incubated for 5 min with serum (15% horse serum and 2.5% fetal bovine serum), human recombinant FGF (100 ng/ml), ACTH (Acthar; 0.1 milliunits/ml) or PMA (0.1 μm) as indicated and then transferred to serum-free medium for 14 h. During the last 2 h of incubation, cells were pulsed with [3H]thymidine (2 μCi/ml). Values for thymidine incorporation and determinations of statistical significance were as described in Table I. Open table in a new tab Cells were growth-arrested as described in Table I, incubated for 5 min with serum (15% horse serum and 2.5% fetal bovine serum), human recombinant FGF (100 ng/ml), ACTH (Acthar; 0.1 milliunits/ml) or PMA (0.1 μm) as indicated and then transferred to serum-free medium for 14 h. During the last 2 h of incubation, cells were pulsed with [3H]thymidine (2 μCi/ml). Values for thymidine incorporation and determinations of statistical significance were as described in Table I. To determine if the cells that were stimulated by ACTH to undergo progression from G1 to S also underwent mitosis, serum-starved Y1 cells were pulsed with ACTH for 5 min and incubated in serum-free medium for an additional 32 h to allow for cell division. Cells were harvested by trypsinization and counted in a hemocytometer. Treatment with ACTH resulted in a 1.5-fold increase in cell number compared with cells maintained over the same period in the absence of ACTH (p = 0.04), thereby demonstrating that short pulses of ACTH are mitogenic. To evaluate the contribution of the cAMP-signaling pathway to the stimulatory effect of ACTH on cell cycle progression, PKA-defective Kin-8 cells were growth-arrested and monitored for incorporation of [3H]thymidine into DNA in the presence of different stimuli. Kin-8 cells responded to a brief pulse of serum as did parental Y1 cells and exhibited a 5- ± 0.5-fold increase in thymidine incorporation (Table II) and a 1.6-fold increase in cell number (p < 0.001). ACTH also stimulated thymidine incorporation into Kin-8 DNA with a 3.4-fold increase that compared favorably with the 4-fold increase seen in parental Y1 cells (Table II) and with a 1.4-fold increase in cell number (p < 0.001) that was not significantly different from the effect of serum. Together, these results indicate that ACTH stimulated adrenal cell proliferation through a cAMP- and PKA-independent mechanism. In both parent Y1 cells and mutant Kin-8 cells, PMA stimulated the transition from G1 to S (Table II), suggesting a possible role for protein kinase C as a mediator of ACTH action. To determine if the stimulatory and inhibitory effects of ACTH on cell cycle progression could be temporally dissociated, labeling indices were determined after incubating serum-starved Y1 cells with FGF together with ACTH added at two different intervals in G1. As shown in Table III, the addition of FGF or ACTH to serum-starved Y1 cells during the first 2 h of G1 increased labeling indices 3.6- and 1.8-fold, respectively, over serum-starved controls. The addition of FGF together with ACTH for the first 2 h of G1 only modestly inhibited the increase in cell cycle progression (38%), whereas the addition of ACTH 2 h after the pulse with FGF inhibited cell cycle progression more dramatically (64%). These observations suggest that ACTH acts at a point early in the G1 phase of the cell cycle to stimulate progression to S phase and acts later in G1 to arrest cell cycle progression.Table IIITemporal effects of ACTH and FGF on cell cycle progression in Y1 adrenal cellsFirst treatmentSecond treatmentLabeled nucleiIncrement over control0–2 h4–6 h%%None9.40.0FGF34.024.6ACTH17.4*8.0*FGF + ACTH24.615.2FGFACTH18.3*8.9*Y1 cells were plated on coverslips, serum-starved for 48 h, and then treated with 100 milliunits/ml ACTH (porcine, Sigma), 20 ng/ml recombinant bovine FGF, or a combination of ACTH + FGF for 2 h as indicated. Cells then were washed and transferred to DMEM without serum and incubated for an additional 22 h. One set of coverslips was treated with FGF for 2 h, transferred to serum-free medium, and challenged with ACTH from the 4th to 6th h of incubation. During the 12–24 h of incubation, all the experimental groups were incubated in serum-free medium containing 100 μm BrdUrd and were then processed to estimate the percentage of nuclei labeled with BrdUrd. Results from three independent experiments were pooled such that 5330–6400 cells were counted per condition. As determined by χ2 analysis with 1 degree of freedom, all differences between means were significant (at < 0.001%) except for the difference between means for the pair marked with asterisks (*). Open table in a new tab Y1 cells were plated on coverslips, serum-starved for 48 h, and then treated with 100 milliunits/ml ACTH (porcine, Sigma), 20 ng/ml recombinant bovine FGF, or a combination of ACTH + FGF for 2 h as indicated. Cells then were washed and transferred to DMEM without serum and incubated for an additional 22 h. One set of coverslips was treated with FGF for 2 h, transferred to serum-free medium, and challenged with ACTH from the 4th to 6th h of incubation. During the 12–24 h of incubation, all the experimental groups were incubated in serum-free medium containing 100 μm BrdUrd and were then processed to estimate the percentage of nuclei labeled with BrdUrd. Results from three independent experiments were pooled such that 5330–6400 cells were counted per condition. As determined by χ2 analysis with 1 degree of freedom, all differences between means were significant (at < 0.001%) except for the difference between means for the pair marked with asterisks (*). c-fos is one of the earliest genes induced by mitogens in G0-arrested fibroblasts and is required for progression of cells through the G1 phase of the cell cycle (29Holt J.T. Gopal T.V. Moulton A.D. Nienhuis A.W. Proc. Natl. Acad. Sci. U. S. A. 1986; 83: 4794-4798Crossref PubMed Scopus (352) Google Scholar, 30Nishikura K. Murray J.M. Mol. Cell. Biol. 1987; 7: 639-649Crossref PubMed Scopus (272) Google Scholar, 31Riabowol K.T. Vosatka R.J. Ziff E.B. Lamb N.J. Feramisco J.R. Mol. Cell. Biol. 1988; 8: 1670-1676Crossref PubMed Scopus (201) Google Scholar, 32Kovary K. Bravo R. Mol. Cell. Biol. 1991; 11: 4466-4472Crossref PubMed Scopus (394) Google Scholar). c-FOS protein was not detected in serum-starved Y1 cells but accumulated with different kinetics after treatment of serum-starved Y1 cells with ACTH, FGF, or fetal bovine serum; after 2 h, c-FOS was detected in at least 80% of nuclei (Fig.5). ACTH and fetal bovine serum caused c-FOS to accumulate more rapidly than did FGF; however, the effects of ACTH and FGF on c-FOS accumulation were sustained over 6 h, whereas the effects of fetal bovine serum were transient and declined after 4 h. The results presented here demonstrate that ACTH, administered to Y1 cells as a short pulse early in the G1 phase of the cell cycle, activates MAP kinase (Fig. 2), promotes the progression of cells from the G1 phase of the cell cycle to S phase (Table IIand Fig. 4), and ultimately stimulates cell division. These effects of ACTH appear to be independent of the cAMP-signaling cascade since ACTH also exerts these effects in the PKA-defective mutant, Kin-8, and may involve a PKC-dependent pathway since these actions of ACTH are mimicked by a pulse of PMA (Fig. 2 and Table II). ACTH is known to activate PKC in Y1 cells (33Widmaier E.P. Hall P.F. Mol. Cell. Endocrinol. 1985; 43: 181-188Crossref PubMed Scopus (46) Google Scholar) 2C. Frigeri and H. A. Armelin, unpublished observations. lending further support to this latter hypothesis. There are, however, other potential pathways through which ACTH may activate the MAP kinase cascade and stimulate cell proliferation. For example, other hormones acting through G protein-coupled receptors have been shown to activate MAP kinasevia a pathway involving activation of G protein β/γ subunits, phosphoinositide 3-kinase, and a src-like tyrosine kinase (34Garnovskaya M.N. van Biesen T. Hawes B. Ramos S.C. Lefkowitz R.J. Raymond J.R. Biochemistry. 1996; 35: 13716-13722Crossref PubMed Scopus (97) Google Scholar, 35Lopez-Ilasaca M. Crespo P. Pellici P.G. Gutkind J.S. Wetzker R. Science. 1997; 275: 394-396Crossref PubMed Scopus (629) Google Scholar). Therefore, the relative contribution of the protein kinase C pathway to the proliferative effects of ACTH has yet to be established. ACTH also stimulates the rapid accumulation of c-FOS protein (Fig. 5). Although not rigorously established in our experiments, results from other systems suggest that this effect of ACTH is likely to be mediated by activation of the MAP kinase-signaling cascade (36Karin M. J. Biol. Chem. 1995; 270: 16483-16486Abstract Full Text Full Text PDF PubMed Scopus (2258) Google Scholar). Indeed, the ACTH-stimulated increase in c-FOS accumulation (Fig. 5) occurs subsequent to the activation of MAP kinase by ACTH (Fig. 2) and also appears to be mediated by a protein kinase C-dependent pathway rather than by a cAMP-dependent mechanism (10Kimura E. Armelin H.A. J. Biol. Chem. 1990; 265: 3518-3521Abstract Full Text PDF PubMed Google Scholar, 11Kimura E. Sonobe M.H. Armelin M.C.S. Armelin H.A. Mol. Endocrinol. 1993; 7: 1463-1471Crossref PubMed Scopus (0) Google Scholar). The observations that ACTH and serum stimulate c-FOS accumulation more rapidly than does FGF (Fig. 5) is also consistent with this hypothesis since ACTH and serum activate the MAP kinase cascade more rapidly than does FGF (Fig. 1). 3B. P. Schimmer and Z. Todorovic, unpublished observations. The transient effect of serum on c-FOS accumulation (Fig. 5) suggests the presence of specific serum factors that control either the stability of the c-fos transcript or c-FOS protein but which appear not to have an impact on the generation of the mitogenic signal. Although Y1 cells are of tumor origin, the cell line behaves in many other respects like normal adrenocortical cells and has long been used as a model adrenocortical cell system (37Schimmer B.P. Sato G. Functionally Differentiated Cell Lines. Alan R. Liss, Inc., New York1981: 61-92Google Scholar, 38Schimmer B.P. Gupta R.S. Drug Resistance in Mammalian Cells. 1. CRC Press, Inc., Boca Raton, FL1989: 185-210Google Scholar). Thus, the finding that ACTH under appropriate circumstances can stimulate the proliferation of Y1 adrenal cells may be physiologically relevant. The results presented here suggest that the trophic effects of ACTH on the adrenal cortex may result from a direct mitogenic effect of the hormone rather than from an indirect effect as postulated previously (2Hornsby P.J. Endocr. Res. 1985; 10: 259-281Crossref Scopus (49) Google Scholar). In addition, our results provide a rationale for the inductive effects of ACTH on genes associated with cell proliferation such as ornithine decarboxylase (9Kudlow J.E. Rae P.A. Gutmann N.S. Schimmer B.P. Burrow G.N. Proc. Natl. Acad. Sci. U. S. A. 1980; 77: 2767-2771Crossref PubMed Scopus (32) Google Scholar) and fos and jun protooncogenes (Fig. 5 and Refs.10Kimura E. Armelin H.A. J. Biol. Chem. 1990; 265: 3518-3521Abstract Full Text PDF PubMed Google Scholar, 11Kimura E. Sonobe M.H. Armelin M.C.S. Armelin H.A. Mol. Endocrinol. 1993; 7: 1463-1471Crossref PubMed Scopus (0) Google Scholar, 12Viard I. Penhoat A. Ouali R. Langlois D. Begeot M. Saez J.M. J. Steroid Biochem. Mol. Biol. 1994; 50: 219-224Crossref PubMed Scopus (12) Google Scholar) that were previously considered to be paradoxical effects of the hormone. Our results also may bear on the controversy regarding the origins of the proliferative cells of the adrenal cortex. According to one hypothesis, proliferative adrenal cells arise from a population of undifferentiated stem cells that expand and differentiate as they move through the distinct zones of the cortex, whereas an alternate hypothesis suggests that proliferative cells arise from differentiated zones of the gland (39Mitani F. Miyamoto H. Mukai K. Ishimura Y. Endocr. Res. 1996; 22: 421-431Crossref PubMed Scopus (33) Google Scholar, 40Holzwarth M.A. Gomez-Sanchez C.E. Engeland W.C. Endocr. Res. 1996; 22: 401-406Crossref PubMed Scopus (6) Google Scholar). Our results that demonstrate proliferative effects of ACTH on a differentiated cell line would seem to be more consistent with the hypothesis that proliferating adrenal cells arise from differentiated zones of the gland rather than from an undifferentiated stem cell population. With prolonged treatment, ACTH inhibits G1 to S progression in Y1 cells (Table I). Our results indicate that the growth-inhibitory effect of ACTH does not result from inhibition of the MAP kinase isoforms Erk1 and Erk2 (Fig. 2). Instead, preliminary results suggest that growth inhibition may result from a posttranscriptional effect of ACTH leading to destabilization of c-myc transcripts (for discussion, see Ref. 8Armelin H.A. Lotfi C.F.P. Lepique A.P. Endocr. Res. 1996; 22: 373-383Crossref PubMed Scopus (19) Google Scholar). Although cAMP analogs and phorbol esters both mimic the growth-inhibiting effect of ACTH, the hormone clearly acts through a cAMP-dependent pathway since the inhibitory effects of ACTH and cAMP analogs are abolished in PKA-defective Kin mutants, whereas the growth-inhibitory effects of phorbol esters persist (7Schimmer B.P. Schulz P. Endocr. Res. 1985; 11: 199-209Crossref PubMed Scopus (24) Google Scholar, 8Armelin H.A. Lotfi C.F.P. Lepique A.P. Endocr. Res. 1996; 22: 373-383Crossref PubMed Scopus (19) Google Scholar).3 The results presented here describe opposing effects of ACTH on Y1 cell proliferation. ACTH administered as a pulse early in the G1phase of the cell cycle stimulates progression to S phase and mitosis, whereas administration of ACTH near the midpoint of G1arrests cell cycle progression (Table III) and is growth inhibitory (7Schimmer B.P. Schulz P. Endocr. Res. 1985; 11: 199-209Crossref PubMed Scopus (24) Google Scholar). On this basis we suggest that the growth-inhibitory effect of ACTH represents a checkpoint in mid-G1 that limits the mitogenic activity of the hormone. We further suggest that a mitogenic response to ACTH must involve other factors that overcome the growth-inhibitory influence of ACTH at or near the midpoint of G1. We thank Jennivine Tsao for excellent technical assistance. Acthar was a gift from Dr. J. Bourgouin (Rhône-Poulenc Rorer, Montreal, Quebec); ACTH1–39was a gift from Dr. K. Scheibli (CIBA-GEIGY, Basel); bovine recombinant basic fibroblast growth factor-2 was a gift from Dr. A. G. Gambarini (Dept. of Biochemistry, University of Sao Paulo).
Referência(s)