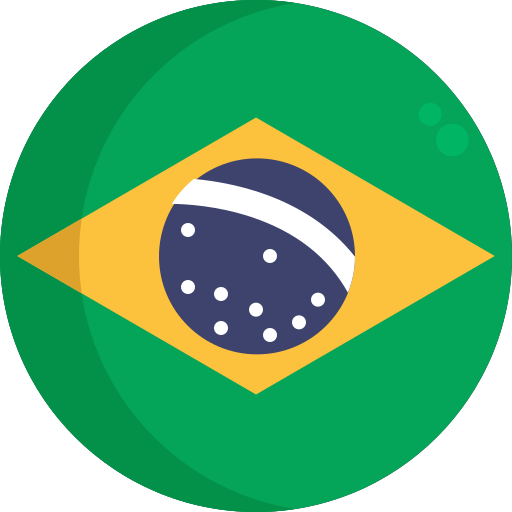
Structural and Biochemical Characterization of Peroxiredoxin Qβ from Xylella fastidiosa
2010; Elsevier BV; Volume: 285; Issue: 21 Linguagem: Inglês
10.1074/jbc.m109.094839
ISSN1083-351X
AutoresBruno Brasil Horta, Marcos Antônio de Oliveira, Karen Fulan Discola, José Renato Rosa Cussiol, Luís Eduardo Soares Netto,
Tópico(s)Insect and Pesticide Research
ResumoThe phytopathogenic bacterium Xylella fastidiosa is the etiological agent of various plant diseases. To survive under oxidative stress imposed by the host, microorganisms express antioxidant proteins, including cysteine-based peroxidases named peroxiredoxins. This work is a comprehensive analysis of the catalysis performed by PrxQ from X. fastidiosa (XfPrxQ) that belongs to a peroxiredoxin class still poorly characterized and previously considered as moderately reactive toward hydroperoxides. Contrary to these assumptions, our competitive kinetics studies have shown that the second-order rate constants of the peroxidase reactions of XfPrxQ with hydrogen peroxide and peroxynitrite are in the order of 107 and 106 m−1 s−1, respectively, which are as fast as the most efficient peroxidases. The XfPrxQ disulfides were only slightly reducible by dithiothreitol; therefore, the identification of a thioredoxin system as the probable biological reductant of XfPrxQ was a relevant finding. We also showed by site-specific mutagenesis and mass spectrometry that an intramolecular disulfide bond between Cys-47 and Cys-83 is generated during the catalytic cycle. Furthermore, we elucidated the crystal structure of XfPrxQ C47S in which Ser-47 and Cys-83 lie ∼12.3 Å apart. Therefore, significant conformational changes are required for disulfide bond formation. In fact, circular dichroism data indicated that there was a significant redox-dependent unfolding of α-helices, which is probably triggered by the peroxidatic cysteine oxidation. Finally, we proposed a model that takes data from this work as well data as from the literature into account. The phytopathogenic bacterium Xylella fastidiosa is the etiological agent of various plant diseases. To survive under oxidative stress imposed by the host, microorganisms express antioxidant proteins, including cysteine-based peroxidases named peroxiredoxins. This work is a comprehensive analysis of the catalysis performed by PrxQ from X. fastidiosa (XfPrxQ) that belongs to a peroxiredoxin class still poorly characterized and previously considered as moderately reactive toward hydroperoxides. Contrary to these assumptions, our competitive kinetics studies have shown that the second-order rate constants of the peroxidase reactions of XfPrxQ with hydrogen peroxide and peroxynitrite are in the order of 107 and 106 m−1 s−1, respectively, which are as fast as the most efficient peroxidases. The XfPrxQ disulfides were only slightly reducible by dithiothreitol; therefore, the identification of a thioredoxin system as the probable biological reductant of XfPrxQ was a relevant finding. We also showed by site-specific mutagenesis and mass spectrometry that an intramolecular disulfide bond between Cys-47 and Cys-83 is generated during the catalytic cycle. Furthermore, we elucidated the crystal structure of XfPrxQ C47S in which Ser-47 and Cys-83 lie ∼12.3 Å apart. Therefore, significant conformational changes are required for disulfide bond formation. In fact, circular dichroism data indicated that there was a significant redox-dependent unfolding of α-helices, which is probably triggered by the peroxidatic cysteine oxidation. Finally, we proposed a model that takes data from this work as well data as from the literature into account. IntroductionThe Gram-negative, xylem-limited phytopathogenic bacterium Xylella fastidiosa is the etiological agent of economically important diseases in citrus and many other plant species. The 9a5c strain of X. fastidiosa, which has been linked to citrus variegated chlorosis, was the first plant pathogenic bacterium whose genome was completely sequenced (1Simpson A.J. Reinach F.C. Arruda P. Abreu F.A. Acencio M. Alvarenga R. Alves L.M. Araya J.E. Baia G.S. Baptista C.S. Barros M.H. Bonaccorsi E.D. Bordin S. Bové J.M. Briones M.R. Bueno M.R. Camargo A.A. Camargo L.E. Carraro D.M. Carrer H. Colauto N.B. Colombo C. Costa F.F. Costa M.C. Costa-Neto C.M. Coutinho L.L. Cristofani M. Dias-Neto E. Docena C. El-Dorry H. Facincani A.P. Ferreira A.J. Ferreira V.C. Ferro J.A. Fraga J.S. França S.C. Franco M.C. Frohme M. Furlan L.R. Garnier M. Goldman G.H. Goldman M.H. Gomes S.L. Gruber A. Ho P.L. Hoheisel J.D. Junqueira M.L. Kemper E.L. Kitajima J.P. Krieger J.E. Kuramae E.E. Laigret F. Lambais M.R. Leite L.C. Lemos E.G. Lemos M.V. Lopes S.A. Lopes C.R. Machado J.A. Machado M.A. Madeira A.M. Madeira H.M. Marino C.L. Marques M.V. Martins E.A. Martins E.M. Matsukuma A.Y. Menck C.F. Miracca E.C. Miyaki C.Y. Monteriro-Vitorello C.B. Moon D.H. Nagai M.A. Nascimento A.L. Netto L.E. Nhani Jr., A. Nobrega F.G. Nunes L.R. Oliveira M.A. de Oliveira M.C. de Oliveira R.C. Palmieri D.A. Paris A. Peixoto B.R. Pereira G.A. Pereira Jr., H.A. Pesquero J.B. Quaggio R.B. Roberto P.G. Rodrigues V. de M Rosa A.J. de Rosa Jr., V.E. de Sá R.G. Santelli R.V. Sawasaki H.E. da Silva A.C. da Silva A.M. da Silva F.R. da Silva Jr., W.A. da Silveira J.F. Silvestri M.L. Siqueira W.J. de Souza A.A. de Souza A.P. Terenzi M.F. Truffi D. Tsai S.M. Tsuhako M.H. Vallada H. Van Sluys M.A. Verjovski-Almeida S. Vettore A.L. Zago M.A. Zatz M. Meidanis J. Setubal J.C. Nature. 2000; 406: 151-159Crossref PubMed Scopus (719) Google Scholar). Citrus variegated chlorosis is the major problem faced by the Brazilian citrus industry and is responsible for significant losses in orange production (2Amaro A.A. Maia M.L. Gonzales M.A. Donadio L.C. Moreira C.S. Citrus Variegated Chlorosis. Estação Experimental de Citricultura, Bebedouro, SP, Brazil1998: 123-139Google Scholar). X. fastidiosa also imposes severe economic damage for other countries of the American continent because other strains of this bacterium provoke Pierce disease in grapevines, phony peach disease, and leaf scorch diseases in almond and oleander (3Hendson M. Purcell A.H. Chen D. Smart C. Guilhabert M. Kirkpatrick B. Appl. Environ. Microbiol. 2001; 67: 895-903Crossref PubMed Scopus (130) Google Scholar). During infection by microorganisms, one of the first plant responses is the production of oxidants, including hydrogen peroxide, organic hydroperoxides, and peroxynitrite (4Wrzaczek M. Brosché M. Kollist H. Kangasjärvi J. Proc. Natl. Acad. Sci. U.S.A. 2009; 106: 5412-5417Crossref PubMed Scopus (53) Google Scholar, 5Tenhaken R. Levine A. Brisson L.F. Dixon R.A. Lamb C. Proc. Natl. Acad. Sci. U.S.A. 1995; 92: 4158-4163Crossref PubMed Scopus (346) Google Scholar, 6Koszelak-Rosenblum M. Krol A.C. Simmons D.M. Goulah C.C. Wroblewski L. Malkowski M.G. J. Biol. Chem. 2008; 283: 24962-24971Abstract Full Text Full Text PDF PubMed Scopus (23) Google Scholar). To counteract the release of these oxidants, microorganisms have developed defenses such as antioxidant enzymes. In the X. fastidiosa genome, five genes encode homologous proteins involved in hydroperoxide decomposition: catalase, glutathione peroxidase (GPx), 2The abbreviations used are: GPxglutathione peroxidaseBcpbacterioferritin comigratory proteinCHPcumene hydroperoxideDTT1,4-dithiothreitolESI-MSelectrospray ionization mass spectrometryHPLChigh performance liquid chromatographyHRPhorseradish peroxidaseNEMN-ethylmaleimidePrxperoxiredoxinTBHPt-butyl hydroperoxideTCEPtris(2-carboxyethyl)phosphineTrxthioredoxinTrxRthioredoxin reductasePDBProtein Data Bank. Ohr, AhpC, and PrxQ proteins (1Simpson A.J. Reinach F.C. Arruda P. Abreu F.A. Acencio M. Alvarenga R. Alves L.M. Araya J.E. Baia G.S. Baptista C.S. Barros M.H. Bonaccorsi E.D. Bordin S. Bové J.M. Briones M.R. Bueno M.R. Camargo A.A. Camargo L.E. Carraro D.M. Carrer H. Colauto N.B. Colombo C. Costa F.F. Costa M.C. Costa-Neto C.M. Coutinho L.L. Cristofani M. Dias-Neto E. Docena C. El-Dorry H. Facincani A.P. Ferreira A.J. Ferreira V.C. Ferro J.A. Fraga J.S. França S.C. Franco M.C. Frohme M. Furlan L.R. Garnier M. Goldman G.H. Goldman M.H. Gomes S.L. Gruber A. Ho P.L. Hoheisel J.D. Junqueira M.L. Kemper E.L. Kitajima J.P. Krieger J.E. Kuramae E.E. Laigret F. Lambais M.R. Leite L.C. Lemos E.G. Lemos M.V. Lopes S.A. Lopes C.R. Machado J.A. Machado M.A. Madeira A.M. Madeira H.M. Marino C.L. Marques M.V. Martins E.A. Martins E.M. Matsukuma A.Y. Menck C.F. Miracca E.C. Miyaki C.Y. Monteriro-Vitorello C.B. Moon D.H. Nagai M.A. Nascimento A.L. Netto L.E. Nhani Jr., A. Nobrega F.G. Nunes L.R. Oliveira M.A. de Oliveira M.C. de Oliveira R.C. Palmieri D.A. Paris A. Peixoto B.R. Pereira G.A. Pereira Jr., H.A. Pesquero J.B. Quaggio R.B. Roberto P.G. Rodrigues V. de M Rosa A.J. de Rosa Jr., V.E. de Sá R.G. Santelli R.V. Sawasaki H.E. da Silva A.C. da Silva A.M. da Silva F.R. da Silva Jr., W.A. da Silveira J.F. Silvestri M.L. Siqueira W.J. de Souza A.A. de Souza A.P. Terenzi M.F. Truffi D. Tsai S.M. Tsuhako M.H. Vallada H. Van Sluys M.A. Verjovski-Almeida S. Vettore A.L. Zago M.A. Zatz M. Meidanis J. Setubal J.C. Nature. 2000; 406: 151-159Crossref PubMed Scopus (719) Google Scholar). All of them except GPx protein were identified in the whole cell extract and extracellular fraction of the citrus-isolated strain 9a5c (7Smolka M.B. Martins-de-Souza D. Martins D. Winck F.V. Santoro C.E. Castellari R.R. Ferrari F. Brum I.J. Galembeck E. Della Coletta Filho H. Machado M.A. Marangoni S. Novello J.C. Proteomics. 2003; 3: 224-237Crossref PubMed Scopus (72) Google Scholar).Among the antioxidant proteins of X. fastidiosa, AhpC and PrxQ are members of a very large and ubiquitous family of cysteine-based peroxidases designated as peroxiredoxin (Prx). Prx enzymes are non-heme peroxidases with a catalytic activity toward hydrogen peroxide, peroxynitrite, and various organic hydroperoxides that is endowed by reactive cysteine residues. Prxs are widely distributed and are found in the majority of the pathogenic bacteria. In Escherichia coli, Prx proteins are among the 10 most abundant cellular proteins (8Link A.J. Robison K. Church G.M. Electrophoresis. 1997; 18: 1259-1313Crossref PubMed Scopus (300) Google Scholar), and in other bacteria, Prxs were characterized as species-specific antigens (9Yamaguchi R. Matsuo K. Yamazaki A. Takahashi M. Fukasawa Y. Wada M. Abe C. Infect. Immun. 1992; 60: 1210-1216Crossref PubMed Google Scholar, 10Torian B.E. Flores B.M. Stroeher V.L. Hagen F.S. Stamm W.E. Proc. Natl. Acad. Sci. U.S.A. 1990; 87: 6358-6362Crossref PubMed Scopus (76) Google Scholar, 11O'Toole P.W. Logan S.M. Kostrzynska M. Wadström T. Trust T.J. J. Bacteriol. 1991; 173: 505-513Crossref PubMed Google Scholar).Prxs have been classified into two groups, 2-Cys Prx and 1-Cys Prx, based on the mechanism of catalysis. The 2-Cys Prx group can be further divided into typical 2-Cys Prx and atypical 2-Cys Prx, according to the localization of the additional cysteine involved in catalysis (12Netto L.E. de Oliveira M.A. Monteiro G. Demasi A.P. Cussiol J.R. Discola K.F. Demasi M. Silva G.M. Alves S.V. Faria V.G. Horta B.B. Comp. Biochem. Physiol. C Toxicol. Pharmacol. 2007; 146: 180-193Crossref PubMed Scopus (109) Google Scholar, 13Rhee S.G. Chae H.Z. Kim K. Free Radic. Biol. Med. 2005; 38: 1543-1552Crossref PubMed Scopus (1131) Google Scholar). All groups share a common initial step of catalysis; the oxidation of a conserved reactive cysteine (the so-called peroxidatic cysteine) to a sulfenic acid intermediate (Cys-SOH) with the reduction of the hydroperoxide substrate to the correspondent alcohol. The fate of the sulfenic acid intermediate is distinct among the Prx groups. In typical 2-Cys Prx enzymes, the sulfenic acid reacts with a second cysteine residue located in the C terminus of the other subunit (the so-called resolving cysteine), resulting in the formation of an intermolecular disulfide bond. In contrast, the resolution reaction in atypical 2-Cys Prx enzymes occurs inside the same subunit, resulting in an intramolecular disulfide bond formation. The 1-Cys Prx enzymes do not contain a resolving cysteine, and their sulfenic acid cysteine is stabilized by the active site micro-environment of the polypeptide backbone. The catalytic cycle is completed when disulfide or sulfenic acid is reduced. For most Prxs, this reduction is carried out by Trx (14Poole L.B. Subcell. Biochem. 2007; 44: 61-81Crossref PubMed Scopus (91) Google Scholar).Besides this classification, several others were proposed based on amino acid sequence similarity (15Hofmann B. Hecht H.J. Flohé L. Biol. Chem. 2002; 383: 347-364Crossref PubMed Scopus (766) Google Scholar, 16Trivelli X. Krimm I. Ebel C. Verdoucq L. Prouzet-Mauléon V. Chartier Y. Tsan P. Lauquin G. Meyer Y. Lancelin J.M. Biochemistry. 2003; 42: 14139-14149Crossref PubMed Scopus (32) Google Scholar). Later on, another classification based on both amino acid sequence and structural similarities was proposed and provided insights on the evolution of proteins within the Trx superfamily, which includes Prxs (17Copley S.D. Novak W.R. Babbitt P.C. Biochemistry. 2004; 43: 13981-13995Crossref PubMed Scopus (126) Google Scholar). Among the four Prx classes, class 1 is the most ancestral from which the other three classes arose. However, they are the least characterized class. Here, we will adopt the systems proposed by Copley et al. (17Copley S.D. Novak W.R. Babbitt P.C. Biochemistry. 2004; 43: 13981-13995Crossref PubMed Scopus (126) Google Scholar), and for clarity the relationship between the various classifications is presented in Table 1. Additionally, GPx enzymes are included in Table 1 because these proteins present the Trx fold and also are cysteine-based thiol-dependent peroxidases (18Rouhier N. Jacquot J.P. Free Radic. Biol. Med. 2005; 38: 1413-1421Crossref PubMed Scopus (162) Google Scholar, 19Herbette S. Roeckel-Drevet P. Drevet J.R. FEBS J. 2007; 274: 2163-2180Crossref PubMed Scopus (168) Google Scholar).TABLE 1Classifications for PrxsSubfamilies of PrxsaFirst line, in boldface type, is the subfamily in which XfPrxQ is included.Mechanism of catalysisRepresentative proteinseBcp and TPx are bacterial Prx designations; PrxQ is a bacterial or plant Prx designation; Dot5, Pmp20, Ahp1, Tsa1, and Tsa2 are fungi Prx designations; Prdx1–6 is a mammalian Prx designation; GPx is the glutathione peroxidase designation.Copley et al. (17Copley S.D. Novak W.R. Babbitt P.C. Biochemistry. 2004; 43: 13981-13995Crossref PubMed Scopus (126) Google Scholar)bData are according to both motifs and structural elements.Trivelli et al. (16Trivelli X. Krimm I. Ebel C. Verdoucq L. Prouzet-Mauléon V. Chartier Y. Tsan P. Lauquin G. Meyer Y. Lancelin J.M. Biochemistry. 2003; 42: 14139-14149Crossref PubMed Scopus (32) Google Scholar)cData are according to conserved residues around the active site: A-type Prx (YPXDF(T/S)]FVCPP(T/S)E(I/L/V)); B-type Prx (HPXDFTPVCPTTE(L/F)); C-type Prx (YPX(A/D)XTP(G/V)CPTX(Q/E)XCX(F/L)); D-type Prx (XP(G/A)A(F/Y)(T/S)(P/G)XCP(S/T)XXHXP); and E-type Prx (XP(D/S)DTXVCPXX(Q/S)X(K/R)). D-type Prx also includes the so-called type II Prxs.Hofmann et al. (15Hofmann B. Hecht H.J. Flohé L. Biol. Chem. 2002; 383: 347-364Crossref PubMed Scopus (766) Google Scholar)dData are according to the five major molecular clades of a dendrogram of the Prx family.Class 1 PrxC-typeVAtypical 2-Cys PrxBcp/PrxQ/Dot5Class 2 PrxE-typeIVAtypical 2-Cys PrxTPxClass 3 PrxD-typeIIIAll of themPrdx5/Pmp20/Ahp1Class 4 PrxA-typeITypical 2-Cys PrxPrdx1–4/Tsa1/Tsa2B-typeII1-Cys PrxPrdx6/Prx1Class 5 PrxfGPx cannot be included into the classifications for Prxs presented above, however they were previously considered as the fifth Prx class (18, 19).Atypical 2-Cys PrxGPxa First line, in boldface type, is the subfamily in which XfPrxQ is included.b Data are according to both motifs and structural elements.c Data are according to conserved residues around the active site: A-type Prx (YPXDF(T/S)]FVCPP(T/S)E(I/L/V)); B-type Prx (HPXDFTPVCPTTE(L/F)); C-type Prx (YPX(A/D)XTP(G/V)CPTX(Q/E)XCX(F/L)); D-type Prx (XP(G/A)A(F/Y)(T/S)(P/G)XCP(S/T)XXHXP); and E-type Prx (XP(D/S)DTXVCPXX(Q/S)X(K/R)). D-type Prx also includes the so-called type II Prxs.d Data are according to the five major molecular clades of a dendrogram of the Prx family.e Bcp and TPx are bacterial Prx designations; PrxQ is a bacterial or plant Prx designation; Dot5, Pmp20, Ahp1, Tsa1, and Tsa2 are fungi Prx designations; Prdx1–6 is a mammalian Prx designation; GPx is the glutathione peroxidase designation.f GPx cannot be included into the classifications for Prxs presented above, however they were previously considered as the fifth Prx class (18Rouhier N. Jacquot J.P. Free Radic. Biol. Med. 2005; 38: 1413-1421Crossref PubMed Scopus (162) Google Scholar, 19Herbette S. Roeckel-Drevet P. Drevet J.R. FEBS J. 2007; 274: 2163-2180Crossref PubMed Scopus (168) Google Scholar). Open table in a new tab Historically, all classes of Prxs have been considered only moderately reactive because their catalytic efficiencies (kcat/Km) toward hydroperoxides as determined by steady-state kinetics were in the 105 m−1 s−1 range. In contrast, selenocysteine-containing GPx (∼108 m−1 s−1) and heme-containing catalases (∼106 m−1 s−1) presented considerably higher values (20Wood Z.A. Schröder E. Robin Harris J. Poole L.B. Trends Biochem. Sci. 2003; 28: 32-40Abstract Full Text Full Text PDF PubMed Scopus (2095) Google Scholar). More recently with the development of new assays, Prx enzymes were considered as reactive as selenium- and heme-containing proteins (21Parsonage D. Youngblood D.S. Sarma G.N. Wood Z.A. Karplus P.A. Poole L.B. Biochemistry. 2005; 44: 10583-10592Crossref PubMed Scopus (171) Google Scholar, 22Ogusucu R. Rettori D. Munhoz D.C. Netto L.E. Augusto O. Free Radic. Biol. Med. 2007; 42: 326-334Crossref PubMed Scopus (157) Google Scholar, 23Trujillo M. Ferrer-Sueta G. Thomson L. Flohé L. Radi R. Subcell. Biochem. 2007; 44: 83-113Crossref PubMed Scopus (112) Google Scholar). However, it should be mentioned that only class 3 Prx and class 4 Prx (composed mostly of typical 2-Cys Prx but also 1-Cys Prx proteins) were analyzed by these assays, and consequently, the catalytic efficiencies for enzymes of the other Prx classes remain to be determined.Class 1 Prx (Table 1) include, among other proteins, Bcp and PrxQ proteins with homologs present in a wide range of organisms from bacteria to unicellular eukaryotes and plants (24Jeong W. Cha M.K. Kim I.H. J. Biol. Chem. 2000; 275: 2924-2930Abstract Full Text Full Text PDF PubMed Scopus (177) Google Scholar, 25Wakita M. Masuda S. Motohashi K. Hisabori T. Ohta H. Takamiya K. J. Biol. Chem. 2007; 282: 27792-27801Abstract Full Text Full Text PDF PubMed Scopus (22) Google Scholar, 26Verdoucq L. Vignols F. Jacquot J.P. Chartier Y. Meyer Y. J. Biol. Chem. 1999; 274: 19714-19722Abstract Full Text Full Text PDF PubMed Scopus (197) Google Scholar, 27Kong W. Shiota S. Shi Y. Nakayama H. Nakayama K. Biochem. J. 2000; 351: 107-114Crossref PubMed Scopus (75) Google Scholar, 28Rouhier N. Gelhaye E. Gualberto J.M. Jordy M.N. De Fay E. Hirasawa M. Duplessis S. Lemaire S.D. Frey P. Martin F. Manieri W. Knaff D.B. Jacquot J.P. Plant Physiol. 2004; 134: 1027-1038Crossref PubMed Scopus (146) Google Scholar). Among all Prxs, proteins belonging to class 1 can be considered the least reactive Prxs toward hydroperoxides (23Trujillo M. Ferrer-Sueta G. Thomson L. Flohé L. Radi R. Subcell. Biochem. 2007; 44: 83-113Crossref PubMed Scopus (112) Google Scholar, 29Park S.G. Cha M.K. Jeong W. Kim I.H. J. Biol. Chem. 2000; 275: 5723-5732Abstract Full Text Full Text PDF PubMed Scopus (199) Google Scholar). Despite this, the biological importance of these proteins comes from the observations that bcp deletion in E. coli rendered cells hypersensitive to hydrogen peroxide, TBHP, and linoleic acid hydroperoxide (24Jeong W. Cha M.K. Kim I.H. J. Biol. Chem. 2000; 275: 2924-2930Abstract Full Text Full Text PDF PubMed Scopus (177) Google Scholar), and expression of prxq in Arabidopsis thaliana was stimulated by hydrogen peroxide, TBHP, and diamide (30Horling F. Lamkemeyer P. König J. Finkemeier I. Kandlbinder A. Baier M. Dietz K.J. Plant Physiol. 2003; 131: 317-325Crossref PubMed Scopus (244) Google Scholar). Interestingly, bcp expression was induced in the bacterium Frankia sp. during the formation of symbiosis with the plant, Alnus glutinosa (31Hammad Y. Maréchal J. Cournoyer B. Normand P. Domenach A.M. Can. J. Microbiol. 2001; 47: 541-547Crossref PubMed Google Scholar). Recently, PrxQ proteins were further categorized into two subfamilies as follows: PrxQα proteins that have the two catalytic cysteine residues (peroxidatic and resolving cysteines) with four-residue spacing, and PrxQβ proteins that do not have a cysteine vicinal to the peroxidatic cysteine (25Wakita M. Masuda S. Motohashi K. Hisabori T. Ohta H. Takamiya K. J. Biol. Chem. 2007; 282: 27792-27801Abstract Full Text Full Text PDF PubMed Scopus (22) Google Scholar).Here, we structurally and functionally characterized a class 1 Prx enzyme from X. fastidiosa named here as XfPrxQ, including the evaluation of its kinetic properties by means of new methodologies currently available. This study represents the first determination of the second-order rate constants for the reaction of a class 1 Prx enzyme with hydrogen peroxide and peroxynitrite. Through this analysis, it was possible to observe that XfPrxQ reactivity toward hydroperoxides is comparable with the most reactive enzymes (class 3 Prx, class 4 Prx, GPx, and catalases). Furthermore, the catalytic cycle of XfPrxQ was elucidated by multiple approaches such as x-ray crystallography, CD, site-directed mutagenesis, biochemical assays, and mass spectrometry. Moreover, by the identification of Trx, among other thiols, as the reducing substrate, we were able to reconstitute in vitro the pathway involved in hydroperoxide decomposition. Finally, we propose a model for the redox-dependent structural changes in PrxQβ proteins that is consistent with all of the data presented here as well as information from the literature.DISCUSSIONClass 1 Prx enzymes (Table 1) are poorly characterized, with crystal structures being elucidated only very recently. This study represents a comprehensive structural and functional analysis of XfPrxQ as a representative enzyme of class 1 Prx. Of the four cysteine residues of XfPrxQ (Cys-23, Cys-47, Cys-83, and Cys-101), three of them are conserved among PrxQβ orthologs (Cys-47, Cys-83, and Cys-101). Here, we unequivocally established that peroxidatic Cys-47 and resolving Cys-83 form an intramolecular disulfide bond in the oxidized state.Functional studies with XfPrxQ mutant proteins indicated the role of Cys-47 as the peroxidatic cysteine. Additional approaches were necessary to identify Cys-83 as the resolving cysteine of XfPrxQ, including mass spectrometry approaches (Fig. 4 and supplemental Table SII). This study is the first experimental demonstration of intramolecular disulfide bond formation in solution by a protein member of the PrxQβ subfamily, providing unequivocal evidence that XfPrxQ follows the atypical 2-Cys Prx mechanism.Compared with class 4 Prx from X. fastidiosa (XfAhpC), high concentrations of thiol compound (2 mm) were required to detect DTT-dependent peroxidase activity of XfPrxQ, suggesting that disulfides in class 1 Prx are very stable. Again, this finding is consistent with the proposal that the recycling step of Prx reduction limits the catalytic cycle (21Parsonage D. Youngblood D.S. Sarma G.N. Wood Z.A. Karplus P.A. Poole L.B. Biochemistry. 2005; 44: 10583-10592Crossref PubMed Scopus (171) Google Scholar, 22Ogusucu R. Rettori D. Munhoz D.C. Netto L.E. Augusto O. Free Radic. Biol. Med. 2007; 42: 326-334Crossref PubMed Scopus (157) Google Scholar, 23Trujillo M. Ferrer-Sueta G. Thomson L. Flohé L. Radi R. Subcell. Biochem. 2007; 44: 83-113Crossref PubMed Scopus (112) Google Scholar, 51Trujillo M. Clippe A. Manta B. Ferrer-Sueta G. Smeets A. Declercq J.P. Knoops B. Radi R. Arch. Biochem. Biophys. 2007; 467: 95-106Crossref PubMed Scopus (133) Google Scholar). This result can explain why DTT-dependent peroxidase activity of XfPrxQ C83S was higher than wild-type XfPrxQ activity (Fig. 3A) even though they have similar second-order rate constants of the reaction with hydrogen peroxide (Fig. 6, A and B). It could also explain, for example, why DOT5 (nuclear thiol peroxidase from Saccharomyces cerevisiae), a class 1 Prx protein, has a markedly lower DTT-dependent antioxidant activity of glutamine synthetase protection than those of the other yeast isoenzymes belonging to class 3 Prx and 4 Prx (29Park S.G. Cha M.K. Jeong W. Kim I.H. J. Biol. Chem. 2000; 275: 5723-5732Abstract Full Text Full Text PDF PubMed Scopus (199) Google Scholar).Therefore, it was relevant to identify in this work that the Trx system of X. fastidiosa composed by XfTsnC and XfTrxR, but not other thiol-reducing systems, is the biological reducing system of XfPrxQ (Fig. 3 and supplemental Figs. S3 and S4). In agreement with this finding, DTT-dependent peroxidase activities of other class 1 Prx enzymes were also greatly stimulated by Trx proteins (25Wakita M. Masuda S. Motohashi K. Hisabori T. Ohta H. Takamiya K. J. Biol. Chem. 2007; 282: 27792-27801Abstract Full Text Full Text PDF PubMed Scopus (22) Google Scholar, 28Rouhier N. Gelhaye E. Gualberto J.M. Jordy M.N. De Fay E. Hirasawa M. Duplessis S. Lemaire S.D. Frey P. Martin F. Manieri W. Knaff D.B. Jacquot J.P. Plant Physiol. 2004; 134: 1027-1038Crossref PubMed Scopus (146) Google Scholar, 56König J. Lotte K. Plessow R. Brockhinke A. Baier M. Dietz K.J. J. Biol. Chem. 2003; 278: 24409-24420Abstract Full Text Full Text PDF PubMed Scopus (153) Google Scholar, 60Lamkemeyer P. Laxa M. Collin V. Li W. Finkemeier I. Schöttler M.A. Holtkamp V. Tognetti V.B. Issakidis-Bourguet E. Kandlbinder A. Weis E. Miginiac-Maslow M. Dietz K.J. Plant J. 2006; 45: 968-981Crossref PubMed Scopus (141) Google Scholar, 61Stork T. Laxa M. Dietz M.S. Dietz K.J. Arch. Microbiol. 2009; 191: 141-151Crossref PubMed Scopus (17) Google Scholar). Furthermore, the midpoint potential (−325 mV) of plant PrxQ is in agreement with the necessity of a protein with high reducing power, such as thioredoxin, to support the peroxidase activity of PrxQ enzymes (28Rouhier N. Gelhaye E. Gualberto J.M. Jordy M.N. De Fay E. Hirasawa M. Duplessis S. Lemaire S.D. Frey P. Martin F. Manieri W. Knaff D.B. Jacquot J.P. Plant Physiol. 2004; 134: 1027-1038Crossref PubMed Scopus (146) Google Scholar).Bisubstrate steady-state kinetics analysis of XfPrxQ resulted in catalytic efficiencies in the range of 103 to 104 m−1 s−1 (Table 2), which would indicate that this peroxidase is only moderately reactive when compared with GPx and catalases (20Wood Z.A. Schröder E. Robin Harris J. Poole L.B. Trends Biochem. Sci. 2003; 28: 32-40Abstract Full Text Full Text PDF PubMed Scopus (2095) Google Scholar). Recent studies indicated that class 3 Prx and class 4 Prx proteins (Table 1) are also highly reactive toward hydroperoxides, and the apparent ambiguity between these studies was resolved with the proposal that reduction of Prxs is the rate-limiting step (21Parsonage D. Youngblood D.S. Sarma G.N. Wood Z.A. Karplus P.A. Poole L.B. Biochemistry. 2005; 44: 10583-10592Crossref PubMed Scopus (171) Google Scholar, 22Ogusucu R. Rettori D. Munhoz D.C. Netto L.E. Augusto O. Free Radic. Biol. Med. 2007; 42: 326-334Crossref PubMed Scopus (157) Google Scholar, 23Trujillo M. Ferrer-Sueta G. Thomson L. Flohé L. Radi R. Subcell. Biochem. 2007; 44: 83-113Crossref PubMed Scopus (112) Google Scholar, 51Trujillo M. Clippe A. Manta B. Ferrer-Sueta G. Smeets A. Declercq J.P. Knoops B. Radi R. Arch. Biochem. Biophys. 2007; 467: 95-106Crossref PubMed Scopus (133) Google Scholar). Similarly, by competitive kinetic studies with HRP, the second-order rate constants of XfPrxQ were shown to be high for hydrogen peroxide (in the 107 m−1 s−1 range) and for peroxynitrite (106 m−1 s−1 range). These are the first determinations of second-order rate constants of the reaction of a reduced class 1 Prx enzyme and hydroperoxides, and the results have changed the paradigm that class 1 Prx enzymes present moderate reactivities within the Prx family of enzymes. Instead, these values demonstrate that class 1 Prx proteins are as reactive toward hydroperoxides as class 3 Prx, class 4 Prx, GPx, and catalases (21Parsonage D. Youngblood D.S. Sarma G.N. Wood Z.A. Karplus P.A. Poole L.B. Biochemistry. 2005; 44: 10583-10592Crossref PubMed Scopus (171) Google Scholar, 22Ogusucu R. Rettori D. Munhoz D.C. Netto L.E. Augusto O. Free Radic. Biol. Med. 2007; 42: 326-334Crossref PubMed Scopus (157) Google Scholar, 23Trujillo M. Ferrer-Sueta G. Thomson L. Flohé L. Radi R. Subcell. Biochem. 2007; 44: 83-113Crossref PubMed Scopus (112) Google Scholar, 51Trujillo M. Clippe A. Manta B. Ferrer-Sueta G. Smeets A. Declercq J.P. Knoops B. Radi R. Arch. Biochem. Biophys. 2007; 467: 95-106Crossref PubMed Scopus (133) Google Scholar), which is consistent with the severe phenotypes derived from the deletion of their genes (24Jeong W. Cha M.K. Kim I.H. J. Biol. Chem. 2000; 275: 2924-2930Abstract Full Text Full Text PDF PubMed Scopus (177) Google Scholar, 30Horling F. Lamkemeyer P. König J. Finkemeier I. Kandlbinder A. Baier M. Dietz K.J. Plant Physiol. 2003; 131: 317-325Crossref PubMed Scopus (244) Google Scholar, 31Hammad Y. Maréchal J. Cournoyer B. Normand P. Domenach A.M. Can. J. Microbiol. 2001; 47: 541-547Crossref PubMed Google Scholar). The second-order rate constant of XfPrxQ reacting with peroxynitrite is similar to the rate constant values of class 4 Prx from bacteria and tryparedoxin peroxidases from trypanosomatids (62Bryk R. Griffin P. Nathan C. Nature. 2000; 407: 211-215Crossref PubMed Scopus (563) Google Scholar, 63Trujillo M. Budde H. Piñeyro M.D. Stehr M. Robello C. Flohé L. Radi R. J. Biol. Chem. 2004; 279: 34175-34182Abstract Full Text Full Text PDF PubMed Scopus (112) Google Scholar) and is even higher than the rate constant values of class 4 Prx from yeast (k ∼105 m−1 s−1) (22Ogusucu R
Referência(s)