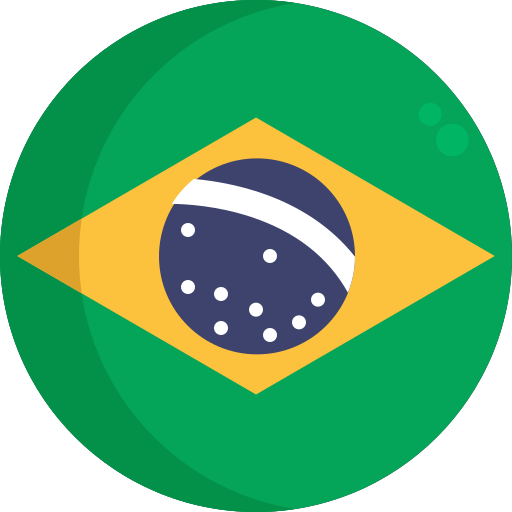
The Astrobiology Primer v2.0
2016; Mary Ann Liebert, Inc.; Volume: 16; Issue: 8 Linguagem: Inglês
10.1089/ast.2015.1460
ISSN1531-1074
AutoresS. D. Domagal-Goldman, Katherine E. Wright, Katarzyna Adamala, Leigh Arina de la Rubia, J. C. Bond, Lewis Dartnell, Aaron D. Goldman, Kennda Lynch, Marie-Ève Naud, Ivan G. Paulino‐Lima, Kelsi Singer, Marina Walther-António, Ximena C. Abrevaya, R. Anderson, Giada Arney, Dimitra Atri, Armando Azúa-Bustos, Jeff S. Bowman, William J Brazelton, G. A. Brennecka, R. Carns, Aditya Chopra, Jesse R. Colangelo-Lillis, Christopher Crockett, Julia DeMarines, Elizabeth A. Frank, Carie M. Frantz, E. De la Fuente, Douglas Galante, Jennifer B. Glass, Damhnait Gleeson, Christopher R Glein, Colin Goldblatt, Rachel Horak, Lev Horodyskyj, Betül Kaçar, Ákos Keresztúri, Emily Knowles, P. A. Mayeur, Shawn E. McGlynn, Yamila Miguel, Michelle Montgomery, C. D. Neish, Lena Noack, Sarah Rugheimer, Eva E. Stüeken, Paulina Tamez-Hidalgo, Sara Imari Walker, Teresa Wong,
Tópico(s)Astro and Planetary Science
ResumoAstrobiologyVol. 16, No. 8 Education ArticleOpen AccessThe Astrobiology Primer v2.0is corrected byCorrection to: Astrobiology 2016;16(8):561–653Co-Lead Editors Shawn D. Domagal-Goldman, Katherine E. Wright, Chapter Editors Shawn D. Domagal-Goldman (Co-Lead Editor, Co-Editor Chapter 1, and Author), Katherine E. Wright (Co-Lead Editor, Co-Editor Chapter 1, and Author), Katarzyna Adamala (Co-Editor Chapter 3 and Author), Leigh Arina de la Rubia (Editor Chapter 9 and Author), Jade Bond (Co-Editor Chapter 3 and Author), Lewis R. Dartnell (Co-Editor Chapter 7 and Author), Aaron D. Goldman (Editor Chapter 2 and Author), Kennda Lynch (Co-Editor Chapter 5 and Author), Marie-Eve Naud (Co-Editor Chapter 7 and Author), Ivan G. Paulino-Lima (Editor Chapter 8 and Author), Kelsi Singer (Co-Editor Chapter 5, Editor Chapter 6, and Author), Marina Walther-Antonio (Editor Chapter 4 and Author), Authors Ximena C. Abrevaya, Rika Anderson, Giada Arney, Dimitra Atri, Armando Azúa-Bustos, Jeff S. Bowman, William J. Brazelton, Gregory A. Brennecka, Regina Carns, Aditya Chopra, Jesse Colangelo-Lillis, Christopher J. Crockett, Julia DeMarines, Elizabeth A. Frank, Carie Frantz, Eduardo de la Fuente, Douglas Galante, Jennifer Glass, Damhnait Gleeson, Christopher R. Glein, Colin Goldblatt, Rachel Horak, Lev Horodyskyj, Betül Kaçar, Akos Kereszturi, Emily Knowles, Paul Mayeur, Shawn McGlynn, Yamila Miguel, Michelle Montgomery, Catherine Neish, Lena Noack, Victoria Petryshyn, Sarah Rugheimer, Eva E. Stüeken, Paulina Tamez-Hidalgo, Sara Imari Walker, and Teresa WongCo-Lead Editors Shawn D. Domagal-GoldmanSearch for more papers by this author, Katherine E. WrightSearch for more papers by this author, Chapter Editors Shawn D. Domagal-Goldman (Co-Lead Editor, Co-Editor Chapter 1, and Author)Address correspondence to: Shawn D. Domagal-Goldman, Planetary Environments Laboratory, NASA Goddard Space Flight Center, 8800 Greenbelt Road, Mail Stop 699.0, Washington, MD 20771, USA E-mail Address: shawn.goldman@nasa.gov*These two authors contributed equally to the work.Search for more papers by this author, Katherine E. Wright (Co-Lead Editor, Co-Editor Chapter 1, and Author)*These two authors contributed equally to the work.Search for more papers by this author, Katarzyna Adamala (Co-Editor Chapter 3 and Author)Search for more papers by this author, Leigh Arina de la Rubia (Editor Chapter 9 and Author)Search for more papers by this author, Jade Bond (Co-Editor Chapter 3 and Author)Search for more papers by this author, Lewis R. Dartnell (Co-Editor Chapter 7 and Author)Search for more papers by this author, Aaron D. Goldman (Editor Chapter 2 and Author)Search for more papers by this author, Kennda Lynch (Co-Editor Chapter 5 and Author)Search for more papers by this author, Marie-Eve Naud (Co-Editor Chapter 7 and Author)Search for more papers by this author, Ivan G. Paulino-Lima (Editor Chapter 8 and Author)Search for more papers by this author, Kelsi Singer (Co-Editor Chapter 5, Editor Chapter 6, and Author)Search for more papers by this author, Marina Walther-Antonio (Editor Chapter 4 and Author)Search for more papers by this author, Authors Ximena C. AbrevayaInstituto de Astronomía y Física del Espacio (IAFE), UBA—CONICET, Ciudad Autónoma de Buenos Aires, Argentina.Search for more papers by this author, Rika AndersonDepartment of Biology, Carleton College, Northfield, Minnesota, USA.Search for more papers by this author, Giada ArneyUniversity of Washington Astronomy Department and Astrobiology Program, Seattle, Washington, USA.Search for more papers by this author, Dimitra AtriBlue Marble Space Institute of Science, Seattle, Washington, USA.Search for more papers by this author, Armando Azúa-BustosBlue Marble Space Institute of Science, Seattle, Washington, USA.Centro de Investigación Biomédica, Universidad Autónoma de Chile, Santiago, Chile.Search for more papers by this author, Jeff S. BowmanLamont-Doherty Earth Observatory, Columbia University, Palisades, New York, USA.Search for more papers by this author, William J. BrazeltonDepartment of Biology, University of Utah, Salt Lake City, Utah, USA.Search for more papers by this author, Gregory A. BrenneckaInstitut für Planetologie, University of Münster, Münster, Germany.Search for more papers by this author, Regina CarnsPolar Science Center, Applied Physics Laboratory, University of Washington, Seattle, Washington, USA.Search for more papers by this author, Aditya ChopraPlanetary Science Institute, Research School of Earth Sciences, Research School of Astronomy and Astrophysics, The Australian National University, Canberra, Australia.Search for more papers by this author, Jesse Colangelo-LillisEarth and Planetary Science, McGill University, and the McGill Space Institute, Montréal, Canada.Search for more papers by this author, Christopher J. CrockettSociety for Science & the Public, Washington, DC, USA.Search for more papers by this author, Julia DeMarinesBlue Marble Space Institute of Science, Seattle, Washington, USA.Search for more papers by this author, Elizabeth A. FrankCarnegie Institute for Science, Washington, DC, USA.Search for more papers by this author, Carie FrantzDepartment of Geosciences, Weber State University, Ogden, Utah, USA.Search for more papers by this author, Eduardo de la FuenteIAM-Departamento de Fisica, CUCEI, Universidad de Guadalajara, Guadalajara, México.Search for more papers by this author, Douglas GalanteBrazilian Synchrotron Light Laboratory, Campinas, Brazil.Search for more papers by this author, Jennifer GlassSchool of Earth and Atmospheric Sciences, Georgia Institute of Technology, Atlanta, Georgia, USA.Search for more papers by this author, Damhnait GleesonScience Foundation Ireland, Dublin, Ireland.Search for more papers by this author, Christopher R. GleinSouthwest Research Institute, San Antonio, Texas, USA.Search for more papers by this author, Colin GoldblattSchool of Earth and Ocean Sciences, University of Victoria, Victoria, Canada.Search for more papers by this author, Rachel HorakAmerican Society for Microbiology, Washington, DC, USA.Search for more papers by this author, Lev HorodyskyjArizona State University, Tempe, Arizona, USA.Search for more papers by this author, Betül KaçarHarvard University, Organismic and Evolutionary Biology, Cambridge, Massachusetts, USA.Search for more papers by this author, Akos KereszturiResearch Centre for Astronomy and Earth Sciences, Hungarian Academy of Sciences, Budapest, Hungary.Search for more papers by this author, Emily KnowlesJohnson & Wales University, Denver, Colorado, USA.Search for more papers by this author, Paul MayeurRensselaer Polytechnic Institute, Troy, New York, USA.Search for more papers by this author, Shawn McGlynnEarth Life Science Institute, Tokyo Institute of Technology, Tokyo, Japan.Search for more papers by this author, Yamila MiguelLaboratoire Lagrange, UMR 7293, Université Nice Sophia Antipolis, CNRS, Observatoire de la Côte d'Azur, Nice, France.Search for more papers by this author, Michelle MontgomeryUniversity of Central Florida, Orlando, Florida, USA.Search for more papers by this author, Catherine NeishDepartment of Earth Sciences, The University of Western Ontario, London, Canada.Search for more papers by this author, Lena NoackRoyal Observatory of Belgium, Brussels, Belgium.Search for more papers by this author, Victoria PetryshynUniversity of Southern California, Los Angeles, California, USA.Search for more papers by this author, Sarah RugheimerDepartment of Astronomy, Harvard University, Cambridge, Massachusetts, USA.University of St. Andrews, St. Andrews, UK.Search for more papers by this author, Eva E. StüekenUniversity of Washington, Seattle, Washington, USA.University of California, Riverside, California, USA.Search for more papers by this author, Paulina Tamez-HidalgoNovozymes A/S, Bagsvaerd, Denmark.Search for more papers by this author, Sara Imari WalkerBlue Marble Space Institute of Science, Seattle, Washington, USA.School of Earth and Space Exploration and Beyond Center for Fundamental Concepts in Science, Arizona State University, Tempe, Arizona, USA.Search for more papers by this author, and Teresa WongDepartment of Earth and Planetary Sciences, Washington University in St. Louis, St. Louis, Missouri, USA.Search for more papers by this authorPublished Online:1 Aug 2016https://doi.org/10.1089/ast.2015.1460AboutSectionsPDF/EPUB Permissions & CitationsPermissionsDownload CitationsTrack CitationsAdd to favorites Back To Publication ShareShare onFacebookTwitterLinked InRedditEmail Table of ContentsChapter 1. Introduction—What Is Astrobiology?Chapter 2. What Is Life?Chapter 3. How Did Earth and Its Biosphere Originate?Chapter 4. How Have Earth and Its Biosphere Evolved?Chapter 5. What Does Life on Earth Tell Us about Habitability?Chapter 6. What Is Known about Potentially Habitable Worlds beyond Earth?Chapter 7. What Are the Signs of Life (Biosignatures) That We Could Use to Look for Life beyond Earth?Chapter 8. What Relevance Does Astrobiology Have to the Future of Life on This Planet?Chapter 9. ResourcesAcknowledgmentsReferencesAbbreviations ListChapter 1. Introduction—What Is Astrobiology?1.1. What is astrobiology?Astrobiology is the science that seeks to understand the story of life in our universe. Astrobiology includes investigation of the conditions that are necessary for life to emerge and flourish, the origin of life, the ways that life has evolved and adapted to the wide range of environmental conditions here on Earth, the search for life beyond Earth, the habitability of extraterrestrial environments, and consideration of the future of life here on Earth and elsewhere. It therefore requires knowledge of physics, chemistry, biology, and many more specialized scientific areas including astronomy, geology, planetary science, microbiology, atmospheric science, and oceanography.However, astrobiology is more than just a collection of different disciplines. In seeking to understand the full story of life in the Universe in a holistic way, astrobiology asks questions that transcend all these individual scientific subjects.Astrobiological research potentially has much broader consequences than simply scientific discovery, as it includes questions that have been of great interest to human beings for millennia (e.g., are we alone?) and raises issues that could affect the way the human race views and conducts itself as a species (e.g., what are our ethical responsibilities to any life discovered beyond Earth?).1.2. Have we already found life beyond Earth?No. There have been many exciting discoveries that suggest life is possible on other planets and moons, but we have not yet detected any definite signs of life beyond Earth. That does not necessarily mean life exists only on Earth, but only that there is not yet compelling evidence for its existence elsewhere. Space missions have explored only a tiny portion of our solar system, and in the few years since we first discovered planets around other stars, the number of such exoplanets known has increased into the hundreds. The search for life beyond Earth has therefore only just begun.Astrobiology is an exciting subject with new ideas that can easily capture the imaginations of both scientists and nonscientists. But like all areas of science, new ideas are subject to detailed scrutiny by the scientific community as part of the quality control process. Only ideas that are considered to be well-supported by evidence are accepted by the scientific community as a whole. This can lead to lively debate within the scientific community and sometimes within the public arena. Whether the new idea is ultimately accepted or not, the process of testing the evidence results in increased knowledge and understanding, not just about the possibility for life beyond Earth but about our own planet as well. Two high-profile announcements within the field of astrobiology illustrate this.In 1996, scientists announced that they had found evidence of fossilized life contained within martian meteorite ALH84001 (McKay et al., 1996). In subsequent years, astrobiologists completed research on the meteorite itself and on the types of "biosignatures" contained within it. As a result, we now know that abiological processes could have created these signals, and the meteorite is not therefore widely considered proof of life beyond Earth (Bradley et al., 1996, 1997; Frankel and Buseck, 2000; Buseck et al., 2001; McKay et al., 2003; Treiman, 2003). For example, one of the "biosignatures" cited by McKay et al. were structures in the meteorite that appeared to resemble fossilized bacteria when viewed under an electron microscope, but later work showed that similar structures can be produced as an artifact of the techniques used to prepare mineral samples for electron microscopy (Bradley et al., 1997). Although the claims made by McKay et al. have not been generally accepted, in the process of testing them astrobiologists advanced research on a variety of topics, including the minimum size of an individual cell and production mechanisms for tiny grains of magnetite. Additionally, astrobiologists were forced to reexamine what constitutes conclusive evidence for past or present life.More recently, a team announced the discovery of a bacterium they claimed could substitute arsenic for phosphorus in its DNA (Wolfe-Simon et al., 2011). These claims have been largely rejected by the scientific community, which has generally concluded that they are not proven by the evidence presented in the paper (Benner, 2011; Borhani, 2011; Cotner and Hall, 2011; Csabai and Szathmáry, 2011; Foster, 2011; Oehler, 2011; Redfield, 2011; Schoepp-Cothenet et al., 2011; Erb et al., 2012; Reaves et al., 2012). Nevertheless, this paper has stimulated a very active debate, and further research will undoubtedly lead to improved understanding of microbial arsenic metabolism. For example, investigations into the claims made by the paper have already led to the hypothesis that the bacteria isolated by Wolfe-Simon et al. may contain a high-affinity phosphorus transporter that is stimulated by arsenic (Foster, 2011).1.3. What is the Astrobiology Primer?The Astrobiology Primer is designed to provide a basic, but comprehensive, introduction to the field of astrobiology. It is longer than a typical review paper but much shorter in length than a textbook, with the goal of being detailed enough to provide a brief overview of the variety of questions investigated by astrobiologists.The Astrobiology Primer is the product of a strong, vibrant, early-career astrobiology community. This is the second version of the Primer, and like the first (Mix et al., 2006), it is a grassroots effort, written by graduate students and postdoctoral researchers. In total, we are a group of 49 authors and editors from 14 different countries. This second edition has been rewritten from scratch. It updates content and is organized around the questions that currently drive research in the field.Our target readers for this document are other early-career astrobiologists, in particular graduate students who are new to the field, but we hope that it will also be useful to a wide range of people for both personal study and teaching.This primer begins with the question of the nature of life (Chapter 2), then goes on to discuss the origins of life and its planetary environment (Chapter 3), the interactions of life with our planet through time (Chapter 4), what we know of habitability from these interactions (Chapter 5), what we know about the habitability of environments outside Earth (Chapter 6), and how we might search for life in those environments (Chapter 7). We close with chapters on the implications of this research for society (Chapter 8) and resources for astrobiologists (Chapter 9).Chapter 2. What Is Life?This simple question is surprisingly difficult to answer yet is fundamental to the success of astrobiological research. Imagine the difficulty of identifying life on other worlds without a clear understanding of what similarities it may or may not share with life on Earth. Or consider the limitations of interpreting possible origin-of-life scenarios without distinguishing between primitive life-forms and the nonliving entities from which they must have emerged. So far, and not without significant effort, no single definition of life has achieved universal acceptance. But the very exercise of attempting to define life reveals and tests its most essential characteristics.2.1. Can we define life?The goal is not to define "life," the word, as it is used and understood in language, but rather to understand "life" as an objective concept that can guide scientific research (Oliver and Perry, 2006). Successful scientific definitions of life attempt to include everything that we already intuitively consider alive and exclude everything that we would not consider alive. For our purposes, such definitions should also be sufficiently broad to include unknown forms of life that independently arose on Earth or elsewhere. If these criteria are satisfied, the definition will be a useful guide in the search for life on other worlds and the study of its origin here on Earth (Ruiz-Mirazo et al., 2004; Oliver and Perry, 2006).This objective, however, is made more difficult to achieve by our lack of a second known instance of life. Because all known life evolved from a single ancestor or ancestral community, every life-form shares a common set of inherited properties (Woese, 1998; Becerra et al., 2007; Theobald, 2010). Consequently, it can be difficult to distinguish general features of life in the Universe from those specific to our own form of life (Gayon, 2010). Cleland and Chyba (2002) were the first to argue that, without additional examples of life and the greater understanding they may provide, it is even impossible to know whether the concept of life describes an objective natural phenomenon or a subjective category that cannot be perfectly defined (Cleland and Chyba, 2002; Robus et al., 2009).Such objective phenomena, which philosophers call "natural kinds," can be defined completely by principles of the natural world without depending on human-made conventions, for example water defined as the molecule H2O. Cleland and Chyba used the term "bachelor," defined as "an unmarried adult human male," to illustrate the sort of category that is not a natural kind. This definition "explain(s) the meaning of (the term) by relating (it) to expressions that we already understand," but the term "bachelor" is not a natural kind in that the terms "adult" and "unmarried" are understood only as cultural concepts, not natural ones. Cleland and Chyba pointed out that "water" was a concept much like this before it became possible to describe water as its molecular formula. Life may be like water, a natural kind waiting for a scientific definition, or may be more like bachelor, a category that can be easily understood but that cannot be defined completely by natural principles.A successful definition of life must not only delineate life and nonlife but also deal with the intermediate stages that may exist between life and nonlife. The origin of life presents just such a test. Our current understanding of life's origin suggests that there were intermediate states through which all forms of life must emerge (Fry, 1995; Luisi, 1998; Perry and Kolb, 2004). In addition, the biosphere today includes entities that may represent intermediate states between life and nonlife. For example, viruses, which some do not consider bona fide organisms, possess many features similar to organisms and may present further evidence that there is a continuum between life and nonlife. All the arguments listed above demonstrate that the goal of creating a clear and objective scientific definition of life is not at all straightforward.2.2. What are the common characteristics of life on Earth?While life on Earth represents only one example, it is the only known example and, therefore, a good place to begin. Any universal characteristic of life on Earth may be universal either because it was inherited from a common origin or because it is a necessary feature of all life in the Universe. The lack of a second example of life frustrates our ability to conclusively differentiate between these two possibilities.The chemistry of life is predominated by only a handful of carbon-based macromolecules common to all organisms: cellular membranes and intracellular compartments are primarily composed of a type of molecule called a "phospholipid," a lipid with a charged phosphate group on one end; genetic information is stored and processed by the nucleic acids DNA and RNA; the catalytic and infrastructural functions of the cell are performed mainly by proteins. Many of these so-called macromolecules are formed through the polymerization of subunits, for example, nucleotides (forming nucleic acids) and amino acids (forming proteins). The cellular pathway that creates proteins from genetic information is also common across life, and the genetic code that translates genetic information into protein molecules is also nearly universal (Knight et al., 2001).In addition to a common biochemistry, all known life-forms exhibit many of the same general traits. Campbell and Reece (2002) listed the following traits that are common to life on Earth: • Ordered structure refers to the high level of organization observed both within cells and within multicellular organisms as well as the bilateral or radial symmetry observed in many organisms.• Reproduction can refer to either the nearly exact duplication of an organism or the production of a new organism through sex between two parent organisms.• Growth and development refers to the processes by which organisms reach maturity, which can take drastically different forms depending on the type of organism.• Energy utilization refers to the capture of energy from sources such as sunlight, inorganic chemical reactions, or organic material produced by other organisms, and subsequent use of that energy for cellular processes and the biosynthesis of cellular components.• Response to the environment refers to the ability of organisms to sense external stimuli and alter their internal environments accordingly.• Homeostasis refers to the maintenance of a steady internal environment regardless of the external environment.• Evolutionary adaptation refers to the process by which populations of organisms adapt to external pressures such as environmental changes through evolution by natural selection.Some phenomena that are clearly not alive demonstrate one or more, but not all, of these features. Crystals, fire, and hurricanes are all able to grow, reproduce, and utilize forms of potential energy. Crystals also demonstrate ordered structure, albeit composed of fewer distinct subunits. Elements of culture, or "memes" as originally coined by Dawkins (1976), reproduce and evolve by selection in ways that are similar to genes (Dawkins, 1976; Dennet, 1995). Many artificial intelligence algorithms are also based on learning by a process similar to evolution by selection (Dennet, 1995).A much more ambiguous example is that of viruses, which are compartmentalized biological elements that contain genomes and reproduce by co-opting the metabolism of an infected cell. Viruses evolve, demonstrate ordered structure, and in some cases undergo a maturation process that could be construed as growth and development. But they cannot reproduce without co-opting the metabolism of their infected hosts. Viruses, themselves, also do not demonstrate homeostasis, energy utilization, or response to the environment. However, many pathogenic bacteria are also unable to reproduce outside a host cell. If complete metabolic autonomy were a requirement for life, animals, plants, and many other forms of life on Earth would not qualify.Viruses are an especially important case for consideration because they are not only similar to cellular organisms in character, but they also use the same chemistry and genetic code that is discussed below. This similarity is necessary because, otherwise, viruses would not be able to take advantage of the host's biosynthetic machinery to reproduce. Several recently discovered viruses have genomes and physical dimensions comparable in size to some cellular organisms and can be infected, themselves, by other viruses (La Scola et al., 2003, 2008).Biological entities smaller than viruses are also capable of evolution by natural selection. For example, transposons are stretches of DNA found in genomes that, at a minimum, encode genes responsible for extracting or copying themselves and inserting themselves or their copies elsewhere in the genome. About 40% of the human genome is composed of transposons (Lander et al., 2001). Such minimal biological agents appear to blur the line between life and nonlife. The line becomes a bit less blurry through the observation that the majority of natural selection in the biosphere acts on genes rather than organisms or populations (Williams, 1966).2.3. So what is life?At a workshop in 2003, every member of the International Society for the Study of the Origin of Life was asked to give his or her definition for life, resulting in 78 different answers (Palyi et al., 2002). Most often, definitions of life draw on one or more of the attributes of life on Earth that were listed above, usually involving some combination of metabolism, reproduction, and evolution. For the reasons described in Section 2.2, no single feature of life is sufficient to define it, as each of these features is shared by other entities that are not generally considered life.In his 1943 lecture series "What is Life?," published in 1944, Erwin Schrodinger introduced several important concepts that would shape future considerations of the question. The first of these is the concept of genetic information or what he called "code-script." At the time of this insight, DNA had not yet been confirmed as the genetic molecule, and its mechanisms of heredity and genetic encoding would not be described until the following decade. A second insight is that life appears to disobey the second law of thermodynamics. That is, the ordered structures of life do not entropically decay toward chemical equilibrium. Life is able to achieve this far-from-equilibrium state by "feeding" on disequilibrium in the environment. We now understand that this maintenance of order is achieved through the coupling of energy metabolism to biosynthetic metabolism.Currently, the most popular definition of life was conceived by a NASA effort to create a "working definition" for their Exobiology and Astrobiology research programs. The result, most often cited as Joyce (1994), defines life as "a self-sustaining chemical system capable of Darwinian evolution." A major strength of this simple and concise definition is that it distinguishes life by the evolutionary process rather than its chemical composition. The definition alludes to biological chemistry through the general term of "self-sustainment." By invoking evolution as a foundational feature of life, the definition also separates the property of life from the characteristics of individual organisms, which are not, themselves, capable of Darwinian evolution. This is because the evolutionary forces discovered by Darwin (e.g., natural selection) operate through the reproductive success of organisms rather than the alteration of individual organisms during their own lifetimes.Critics of the definition find that the stipulation of Darwinian evolution is overly specific (Cleland and Chyba, 2002). They argue that an extraterrestrial life-form may have a genetic system that evolves through changes accumulated during an organism's lifetime (i.e., Lamarckian evolution) rather than Darwinian selection processes. Nevertheless, the NASA/Joyce definition is perhaps the most widely accepted definition of life within the astrobiological research community.2.4. How should we use our concepts of life?Even a less-than-perfect definition of life may be employed in the search for life on other worlds. For example, any "self-sustained chemical system" must differ in composition from its surroundings. Because life must also be "capable of undergoing Darwinian evolution," this will result in successful species that amplify this chemical signature. McKay (2004) proposed that this chemical signature is a universal feature of all life and may be used for life detection. But while the Joyce (1994) definition gives us a useful way to identify candidate life-forms, it also requires that the life-form be verified by no less than clear evidence of evolution (Luisi, 1998; Cleland and Chyba, 2002).A more pragmatic approach, and the only one used for life-detection experiments so far, is to look for signatures of life that are similar to those that would be produced by life on Earth (see Chapter 7). The first such attempts were made by experimental modules on NASA's Viking Mars landers in the mid-1970s (Klein et al., 1976). These attempts focused on the search for the organic molecules of which Earth-based organisms are composed, and experiments designed to detect the production and consumption of gases by any organisms using similar metabolisms to those used by Earth-based organisms. A more recent approach to develop a life-detection experiment is the Life Marker Chip, designed to detect a broad range of biomolecules in either their pristine or degraded states (Parnell et al., 2007). To do so, the Life Marker Chip would consist of an array of antibody proteins that are designed to bind biomolecules common to life on Earth.Scientific research will continue to enhance our understanding of life on Earth and its effect on the planet. Considering which of the traits demonstrated by life on Earth may be universal
Referência(s)