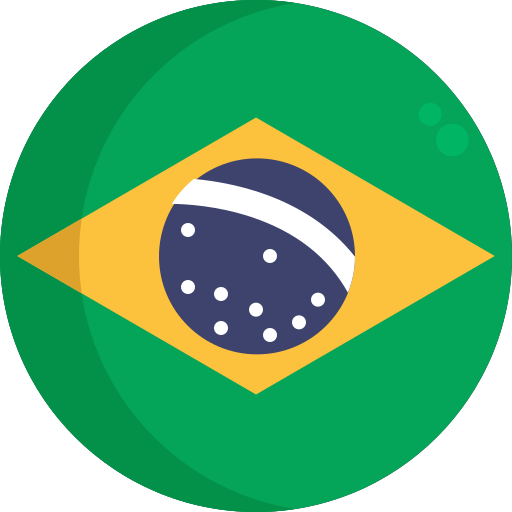
Estimating microbial populations by flow cytometry: Comparison between instruments
2016; Wiley; Volume: 14; Issue: 11 Linguagem: Inglês
10.1002/lom3.10135
ISSN1541-5856
AutoresCatherine Gérikas Ribeiro, Dominique Marie, Adriana Lopes dos Santos, Frederico Pereira Brandini, Daniel Vaulot,
Tópico(s)Marine and coastal ecosystems
ResumoLimnology and Oceanography: MethodsVolume 14, Issue 11 p. 750-758 Evaluations of Existing MethodsFree Access Estimating microbial populations by flow cytometry: Comparison between instruments Catherine Gérikas Ribeiro, Corresponding Author Catherine Gérikas Ribeiro catherine.gerikas@gmail.com Departamento de Oceanografia Biológica, Instituto Oceanográfico, Universidade de São Paulo, São Paulo, BrasilCorrespondence: catherine.gerikas@gmail.comSearch for more papers by this authorDominique Marie, Dominique Marie Sorbonne Universités, UPMC Univ Paris 06, CNRS, UMR 7144, Station Biologique, Place Georges Teissier, Roscoff, FranceSearch for more papers by this authorAdriana Lopes dos Santos, Adriana Lopes dos Santos Sorbonne Universités, UPMC Univ Paris 06, CNRS, UMR 7144, Station Biologique, Place Georges Teissier, Roscoff, FranceSearch for more papers by this authorFrederico Pereira Brandini, Frederico Pereira Brandini Departamento de Oceanografia Biológica, Instituto Oceanográfico, Universidade de São Paulo, São Paulo, BrasilSearch for more papers by this authorDaniel Vaulot, Daniel Vaulot Sorbonne Universités, UPMC Univ Paris 06, CNRS, UMR 7144, Station Biologique, Place Georges Teissier, Roscoff, FranceSearch for more papers by this author Catherine Gérikas Ribeiro, Corresponding Author Catherine Gérikas Ribeiro catherine.gerikas@gmail.com Departamento de Oceanografia Biológica, Instituto Oceanográfico, Universidade de São Paulo, São Paulo, BrasilCorrespondence: catherine.gerikas@gmail.comSearch for more papers by this authorDominique Marie, Dominique Marie Sorbonne Universités, UPMC Univ Paris 06, CNRS, UMR 7144, Station Biologique, Place Georges Teissier, Roscoff, FranceSearch for more papers by this authorAdriana Lopes dos Santos, Adriana Lopes dos Santos Sorbonne Universités, UPMC Univ Paris 06, CNRS, UMR 7144, Station Biologique, Place Georges Teissier, Roscoff, FranceSearch for more papers by this authorFrederico Pereira Brandini, Frederico Pereira Brandini Departamento de Oceanografia Biológica, Instituto Oceanográfico, Universidade de São Paulo, São Paulo, BrasilSearch for more papers by this authorDaniel Vaulot, Daniel Vaulot Sorbonne Universités, UPMC Univ Paris 06, CNRS, UMR 7144, Station Biologique, Place Georges Teissier, Roscoff, FranceSearch for more papers by this author First published: 08 August 2016 https://doi.org/10.1002/lom3.10135Citations: 29AboutSectionsPDF ToolsRequest permissionExport citationAdd to favoritesTrack citation ShareShare Give accessShare full text accessShare full-text accessPlease review our Terms and Conditions of Use and check box below to share full-text version of article.I have read and accept the Wiley Online Library Terms and Conditions of UseShareable LinkUse the link below to share a full-text version of this article with your friends and colleagues. Learn more.Copy URL Share a linkShare onFacebookTwitterLinkedInRedditWechat Abstract For almost three decades, flow cytometry has allowed researchers to investigate ocean planktonic communities using size and cell fluorescence properties. However, oceanographic applications must face two constraints. First, when dealing with marine microbes, instruments must be sensitive because these organisms are very small and with low fluorescence. Second, instruments must be portable to be used on board ships. We compared the performance of two instruments, the BD FACSCanto™ and BD Accuri™ C6. The former is an expensive laboratory-based instrument which has a very good sensitivity, whilst the latter is less sensitive but presents critical advantages for field studies (easy handling and transportation, relatively low cost). We have analyzed 102 samples from the South Atlantic Ocean from three transects off Brazil, within the euphotic zone. We compared cell abundance of heterotrophic bacteria, Prochlorococcus and Synechococcus, as well as photosynthetic pico- and nano-eukaryotes. Heterotrophic bacteria, pico- and nano-eukaryotes could be easily detected with both cytometers. Prochlorococcus and Synechococcus populations were severely under-estimated with the BD Accuri™ C6, particularly for samples from the well-lit layers of the water column. Correction of abundance data using previously suggested approaches was not sufficient to fully compensate for the low sensibility. Our data suggest that the BD Accuri™ C6 is suitable for counting marine bacteria and photosynthetic eukaryotes, but not Prochlorococcus and Synechococcus. Flow cytometry (FCM) is a well-established technique (Marie et al. 1997; Gasol and del Giorgio 2000) used since the 1980s (Trask et al. 1982; Olson et al. 1985) for enumeration and characterization of marine micro-organisms. FCM analysis of planktonic communities fulfills the scientific demands of rapid and accurate cell counting, as it considerably reduces the bias introduced by visual counting (Marie et al. 2005). By simultaneously recording several parameters during analysis, FCM allows the discrimination of pico- and nanoplankton populations and the estimation of their abundance, cell size, and pigment content (Marie et al. 2005), both by natural (chlorophyll, phycoerythrin) or induced (fluorescent dyes) fluorescence (Marie et al. 1997). The flow cytometer registers events as cells are aligned in a fluid stream and flow through a beam of focused light usually provided by one or several lasers. For each particle, scattered light and emitted fluorescence are converted to digital signals and recorded. A flow cytometer comprises three main systems: fluidics (particle transport), optics (laser beam and optical filters), and electronics (signal conversion into electronic data). Detectors for scattered light located at 180° and 90° from the light source are called forward scatter (FSC) and side scatter (SSC), respectively. Fluorescence at different wavelengths (typically green, orange and red) is also recorded. Signals associated with each parameter are displayed as cytograms, which are used to discriminate and count different populations based on scattering and fluorescence features. Phytoplankton populations can be differentiated by FCM according to specific values of the recorded parameters (FSC, SSC, red or orange fluorescence). Bacteria are in general detected after staining with a nucleic acid strain such as SYBR Green-I (Marie et al. 1997). Two different groups can be distinguished based on their apparent nucleic acid content (differences in fluorescence intensity) and side scatter signal (SSC): high nucleic acid (HNA) and low nucleic acid (LNA) bacteria. The function and ecological importance of these two groups is far from being fully understood (Bouvier et al. 2007; Van Wambeke et al. 2011), and several studies have addressed these nucleic acid content differences in terms of ecological traits, such as bacterial activity and production (Morán et al. 2007; Ortega-Retuerta et al. 2008; Van Wambeke et al. 2011). Two main groups of autotrophic prokaryotes dominate picoplankton: Prochlorococcus and Synechococcus. Prochlorococcus is ubiquitous in the euphotic zone of tropical oceans, being considered the most abundant photosynthetic organisms on the planet (Partensky et al. 1999b), and its discovery was only made possible with the development of flow cytometry (Chisholm et al. 1988). Prochlorococcus is discriminated by its small scattering and low red fluorescence (chlorophyll). Synechococcus is widely distributed in marine environments, being particularly abundant in well-lit and nutrient rich top layers of the oceans (Partensky et al. 1999a). One of the key parameters that allows Synechococcus populations to be discriminated by FCM is the phycoerythrin content (orange fluorescence). Different Synechococcus clades can show distinct fluorescence signatures (Olson et al. 1990; Thompson and van den Engh 2016), as a result of different phycobilisome composition (Scanlan et al. 2009). Pico- and nanoeukaryotes are important contributors to global primary productivity (Li 1994), and due to their larger cell size, they often contribute to an important share of autotrophic biomass in the oceans (Zubkov et al. 1998). Picoeukaryotes, which cells range from 0.8 μm to 2–3 μm (Simon et al. 1994), present well-defined cytometric signatures by FCM, while nano-eukaryotes populations are less well defined. FCM analysis has led to numerous advances in marine microbial ecology, although cost and maintenance expenses were prohibitive for many laboratories until recently (Gasol and del Giorgio 2000; Vives-Rego et al. 2000). Since the first cytometry-based field study made by Olson et al. (1985), on-board flow cytometry has become a crucial tool in the investigation of both autotrophic and heterotrophic picoplanktonic communities (Legendre et al. 2001). The manufacturing of low cost compact benchtop flow cytometers such as the BD Accuri™ C6, the Millipore Guava® or the Applied Biosystems Attune® has facilitated the use of FCM to study of phytoplankton communities around the world, due to easy handling, automatic sampling and easy transportation (a critical quality for field measurements). However, these low cost instruments can be less sensitive than laboratory based flow cytometers, due to less sophisticated optical and/or electronic systems. A lower sensitivity is usually not a problem for bacteria which are detected after staining with strongly fluorescing dyes such as SYBR Green, or for small eukaryotes whose pigment content is relatively high. However, this is not the case for cyanobacteria like Prochlorococcus, for which the concentration of photosynthetic pigments per cell is as much as 50–100 fold lower in cells exposed to high light as a result of photo-acclimation (Sosik et al. 1989; Olson et al. 1990), creating “dim” populations in the surface layers. Such low fluorescence explains why Prochlorococcus escaped detection by researchers using epifluorescence microscopy or even during the first use of FCM on board oceanographic ships (Olson et al. 1985). To overcome the problem of low sensitivity flow cytometers, both direct and indirect approaches to infer Prochlorococcus abundance have been developed, such as changes in cytometer optical set up to improve excitation energy or fluorescence detection (Dusenberry and Frankel 1994; Partensky et al. 1999b) and the use of mathematical corrections (Zubkov et al. 1998; Crosbie and Furnas 2001). In this paper, we compare data obtained on marine microbial communities with two flow cytometers, the FACSCanto™ and the Accuri™ C6 (hereafter named as CANTO and C6). Although manufactured by the same company (BD Biosciences, San Jose, CA), these cytometers present distinct fluorescence excitation/detection technical features (Table 1). Differences in laser, optics and electronic systems can potentially affect sensitivity and resolution, influencing the accuracy of field measurements. We analyzed heterotrophic marine bacteria, photosynthetic eukaryotes and cyanobacteria on a set of marine samples from the South Atlantic Ocean (displaying both nutrient and light gradients within the water column). While both instruments produced equivalent data for bacteria and eukaryotes, cyanobacteria, especially Prochlorococcus, were severely under-estimated with the C6 instrument, and procedures previously suggested to correct the data proved ineffective. Table 1. Technical features of BD FACSCanto™ and BD Accuri™ C6 flow cytometers according to the manufacturer. Features BD FACSCanto™ BD Accuri™ C6 Weight 149.7 kg 13.6 kg Acquisition Software BD FACSDiva BD CSampler™ Signal Processing Digital Digital Number of lasers 2 2 Total PMTa for fluorescence 8 4 Laser configuration Blue/red Blue/red Laser wavelength 488 nm, 20 mW solid state 633 nm, 17 mW HeNe 488 nm; 50 mW solid state 640 nm; 30 mW diode Excitation light Optic fiber Direct Florescence sensitivity FITC† < 100 MESFc PEd < 50 MESF FITC < 150 MESF PE < 100 MESF Optical alignment Fixed alignment Fixed alignment Fluidics Positive-pressure pump Peristaltic pump Sample acquisition 18 bits/5 decades 24 bits/7 decades Sample processing Tubes Tubes/96-well plates a PMT: photomultiplier. b FITC: fluorescein isothiocyanate c MESF: molecules of equivalent soluble fluorochrome. d PE: phycoerythrin. Materials and procedures Sampling Water samples were collected onboard the R/V “Alpha Crucis,” between 31 October 2013 and 23 November 2013. The surveyed area was located between latitude 23°11′S–30°52′S and longitude 39°22″W–49°09″W, along three transects (TR1, TR2 and TR3), in the South West Atlantic off Brazil, reaching the 3510 m isobath (Fig. 1). The sampling strategy comprised cross-shelf transects with five depths per station within the euphotic zone for TR1 and TR2, as well as 12 surface samplings for TR3, for a total of 102 samples. Three water masses were sampled during the cruise: the warm and oligotrophic Tropical Water, the cold and nutrient rich South Atlantic Central Water and the Coastal Water, with highly variable features (Castro et al. 2006). Except for TR3 samples, which were collected using a polycarbonate bucket, seawater samples were collected with 12 L Niskin bottles attached to a Seabird® CTD-rosette system (Sea-Bird Electronics, Bellevue, WA), divided into cryotubes, preserved with 0.1% glutaraldehyde, final concentration (modified from Vaulot et al. 1989), incubated for 10 min in the dark, flash-frozen in liquid nitrogen and stored at −80°C until analysis. Figure 1Open in figure viewerPowerPoint Stations sampled in the South Atlantic Ocean off Brazil during the CARBOM cruise in 2013. Profiles: transect 1 (TR1, shaded circles); transect 2 (TR2, shaded triangles) and surface sampling, transect 3 (TR3, shaded squares). The grey scale on the right indicates bottom depths. Flow cytometry analysis Two flow cytometers were used in this study: a BD FACSCanto II™ and a BD Accuri™ C6 (Table 1). Samples were counted simultaneously on both cytometers located in the same room, in order to avoid any possible bias by manipulation or time span between measurements. The tubing of the C6 was new and fluidics were calibrated for precise volume measurements as recommended by the manufacturer (Section 4.13 of manual). Samples were first analyzed unstained to enumerate phototrophs. Fluorescent beads (0.95 G Fluoresbrite® Polysciences, Warrington, PA) were added in each sample to normalize parameters (Marie et al. 1997). A second analysis was performed to enumerate heterotrophic bacteria after staining with SYBR Green® (1:10000, final concentration) (Ref-S7585, Life Technologies, Eugene, Oregon). On the C6, for enumerating phytoplankton, 200 μL of sample were analyzed at the “high” rate (66 μL min−1) with a threshold set at 700 on red fluorescence (FL3-H). To enumerate heterotrophs, 60 μL of SYBR Green stained samples were run at “medium” rate (35 μL min−1) and the threshold was set at 700 on green fluorescence (FL1-H). In both cases, thresholds were determined by running 0.2 μm filtered sea water sample and lowering the values until electrical or optical noise appears. On the CANTO for enumerating phytoplankton, samples were run for 3 min with a rate of 72 μL min−1 and with the discriminator set on red fluorescence at 200. For bacterial enumeration, SYBR Green stained samples were run for 2 min at a rate of 60 μL min−1 and the threshold was set on green fluorescence at 500. Flow rate was determined by the method described by Marie et al. (1997). A known volume of seawater was injected for at least 10 min. Then the remaining volume is measured and the rate is determined by dividing the difference between initial and final volumes by the injection time. Data were analyzed with the Flowing Software® 2.5 (http://www.flowingsoftware.com). Each population was identified on the cytograms on the basis of its scatter and fluorescence signals (Supporting Information Fig. S1). Each parameter was normalized to that of the reference beads (0.95 μm). Cell counts for each red fluorescence value were exported from the single parameter histogram. The resulting spreadsheet (File S1) was used in subsequent analysis with the R software (Team R Development Core 2013). Near the surface the red chlorophyll fluorescence of the picophytoplankton decreases due to photoacclimation (Partensky et al. 1993; Dusenberry et al. 2001; Kulk et al. 2011). Therefore, for a fraction or even all of the Prochlorococcus and Synechococcus populations, fluorescence can fall below the detection threshold (Fig. 2). For the case where only a part of the population was in the noise, we modified the correction procedure described by Crosbie and Furnas (2001) and implemented it as an R routine (File S2). This correction assumes that the red fluorescence distribution of these populations has a log-normal shape (Crosbie and Furnas 2001; Shapiro 2003 1995) and that, when the left part of the distribution is partially in the noise, the left part can be extrapolated from the right part. The R routine takes as input histograms produced by the Flowing Software® (but can be adapted to other data formats) and outputs uncorrected and corrected cell abundance data (output data examples can be found in Files S2 and S3). Three cases can occur for a given population. Figure 2Open in figure viewerPowerPoint Examples of depth profiles (St. 100 and St. 114) of normalized red fluorescence distribution (relative cell number vs. chlorophyll fluorescence) and cell abundance for Prochlorococcus (a–l) and Synechococcus (m–x) on BD FACSCanto™ and BD Accuri™ C6. For each distribution, it is indicated whether the cells were in the noise (Noise) or whether a correction was needed (Corr.). In the depth profiles (f, l, r, x), solid symbols represent samples for which no correction was needed; grey symbols indicate samples for which we applied a correction (see Materials and Methods); samples within noise were removed. The mode of the histogram is not visible (e.g., Fig. 2G). In this case, the population is considered to be mostly within noise, without the possibility of counting or correction. Hence, this population is removed from the dataset and labeled as “cells in noise” by the R routine. The mode is visible, but the left part of the distribution is partly below the noise level (e.g., Fig. 2A). In this case, the abundance of each population is calculated as the double of the right part of the histogram, from the mode to the maximum, and samples are labeled as “correction” The mode is visible and the distribution is totally out of the noise (e.g., Fig. 2C). In this case no correction is performed and the initial output value is kept (“no correction” samples). In some cases, the automatic correction needs some degree of visual confirmation, especially for deeper samples with low cell numbers resulting in noisy histograms (e.g., Fig. 2Q or 2W). Therefore, the R routine provides a graphical output of the histogram for each sample (File S3), allowing the user to visually confirm whether the automatic labeling (case 1, 2, or 3 above) is correct. Statistical analyses were performed with the PRISM® 7 software (http://www.graphpad.com/scientific-software/prism). Results Bacterial populations were well resolved for both CANTO and C6 for all samples (Supporting Information Fig. S1). High Nucleic Acid and Low Nucleic Acid bacterial populations were consistently distinguished with both cytometers and there was a very good correlation for both HNA and LNA (R2 = 0.85 and 0.89, respectively) between the data obtained on the different instruments (Fig. 3A,B). For HNA slope was statistically different from 1 (p < 0.0001) and abundances estimated by the C6 were consistently lower by 10–15% compared to the CANTO. Figure 3Open in figure viewerPowerPoint Relationship between abundance measurements performed with BD Accuri™ C6 and BD FACSCanto™ (in cells/mL−1): (a) HNA bacteria; (b) LNA bacteria; (c) Prochlorococcus; (d) Synechococcus; (e) picoeukaryotes, and (f) nanoeukaryotes. For Prochlorococcus and Synechococcus: “no correction”: solid circles, “correction”: grey squares; “cells in noise”: open triangles. The coefficient of determination and the equation are indicated on each graphic. The regression line calculated from “no correction” samples is marked in black. All the slopes differed significantly from 1 (p < 0.0001), except for LNA bacteria (p = 0.049). The chlorophyll fluorescence of Prochlorococcus and Synechococcus decreases from the deeper layers to the surface in response to photoacclimation. For samples near the surface, cells from both populations can be partly or totally in the noise depending on the instrument sensitivity. Fig. 2 demonstrates clearly that the C6 is less sensitive than the CANTO by at least a factor of 10 (note for example Fig. 2C,I for Prochlorococcus at 110 m for the CANTO and C6, the mode of the histogram is in the noise for the C6 and about 10 times higher than the noise level for the CANTO). With the C6, Prochlorococcus populations were completely in the noise above 100 m (55 out of 102 samples, Figs. 2, 4, Table 2) and for Synechococcus partly or completely in the noise above 50 m (15 out of 102 samples, Figs. 2, 4, Table 2). With the CANTO, only surface Prochlorococcus were partly in the noise and Synechococcus was always fully resolved (Figs. 2, 4, Table 2). When cells were only partly in the noise (i.e., when the histogram mode was clearly visible, e.g., Fig. 2U), we estimated the part of the population that was in the noise using the approach proposed by Crosbie and Furnas (2001) (see Material and Methods section). The comparison between the C6 data which required correction and the CANTO data that did not require correction allowed us to assess the validity of this approach (Fig. 3C,D). Figure 4Open in figure viewerPowerPoint Vertical abundance distribution (cells/mL−1) for measurements with BD FACSCanto™ (left column) and BD Accuri™ C6 (right column): Prochlorococcus (a, b, e, f) and Synechococcus (c, d, g, h). Top labels correspond to station number. Sampled points are marked as: “no correction” (solid circles), “correction” (grey squares), or “cells in noise” (open triangles). Figures were drawn with the Ocean Data View software (https://odv.awi.de/). Table 2. Number of samples assigned as “correction,” “no correction” and “cells in noise” for each pico-cyanobacteria group and equipment tested. No correction Correction Cells in noise CANTO - Prochlorococcus 74 27 1 C6 - Prochlorococcus 27 20 55 CANTO - Synechococcus 101 1 0 C6 - Synechococcus 58 29 15 Clearly some data points that are corrected (grey squares) appear as outliers and are severely underestimated with the C6, even after correction (Fig. 3C,D). The slopes for non-corrected samples (solid circles) are significantly different from 1 (p < 0.0001), being respectively 0.75 (Prochlorococcus) and 0.77 (Synechococcus) (Fig. 3C,D), which corresponds to 25% lower abundance on average with the C6. Vertical cross sections of two transects (Fig. 4) illustrate that, while the CANTO provides fully resolved vertical profiles for both Prochlorococcus and Synechococcus population, the data from the C6 cannot be used in the upper layer (roughly from 100 m to the surface). Pico- and Nano-eukaryotes were always above the detection limit for both instruments with excellent correlation between the two instruments (R2 = 0.94 and 0.69, respectively, Fig. 3E,F). As for the other populations, the slopes were significantly different from 1 (p < 0.0001). While picoeukaryotes were about 15% more abundant with the C6, it was the reverse for nanoeukaryotes which were slightly underestimated by the C6. Discussion The analysis of planktonic communities by flow cytometry is complex because the distinctive cell features of each population may change with depth, diel cycle and nutrient conditions (e.g., Vaulot and Marie 1999). From the six planktonic groups studied here, four were well resolved by both the CANTO and C6 flow cytometers: HNA and LNA heterotrophic bacteria, autotrophic pico-eukaryotes and nano-eukaryotes. Abundance measured by both instruments were tightly correlated. Abundance was always slightly lower with the C6 than with the CANTO, except for picoeukaryotes. This could have resulted from an imperfect calibration of the analyzed volume for one of the instruments. While on the CANTO, the analyzed volume is manually calibrated, the C6 relies on a calibration every time tubing is changed following the manufacturer's recommendation. However, we have recently observed that the actual volume analyzed varies, even during the course of a day, and needs to be re-calibrated at fixed intervals using a procedure similar to the one used for the CANTO (D. Marie, unpublished data). The slightly lower correlation coefficient observed for nanoeukaryotes could result from the difficulty to clearly distinguish the limits of the pico and nano-eukaryote populations. In contrast, the lower sensitivity of the C6 led a drastic underestimation or even non-detection of the cyanobacteria in the upper 100 m of the water column, especially for Prochlorococcus. But the phenomenon was also present for Synechococcus although it had not been recognized previously. For both populations, corrections, such as those recommended previously (Zubkov et al. 1998; Crosbie and Furnas 2001), did not completely solve the problem, since in some samples the corrected counts were still much lower than those obtained with the CANTO (Fig. 3C,D). The data obtained with the C6 for Prochlorococcus but also Synechococcus, should be considered with great caution even when only a part of the population is in the noise. The oceanographic transects (Fig. 4) demonstrate that the C6 instrument would result in very serious underestimates of the cyanobacteria abundance and therefore contribution to the carbon biomass, at least in oligotrophic to mesotrophic waters. For Synechococcus, one solution could be to trigger acquisition on PE fluorescence (FL2) which is general quite strong, but this will require to run every sample twice, with the trigger on FL2 and FL3 respectively. Unfortunately this was not tested on our samples. For Prochlorococcus, the only solution to overcome the problem of low sensitivity is to increase either the excitation energy or the fluorescence detection through optical solutions (Dusenberry and Frankel 1994; Partensky et al. 1999b). Conclusions The increasing affordability of benchtop flow cytometers comes with limitations in some of the equipment features, such as lower detection limits. The comparison between studies of phytoplankton communities by flow cytometry should take into account the equipment used, particularly in approaches involving populations of Prochlorococcus and Synechococcus, in which low chlorophyll concentration per cell can lead to the underestimation of their abundance in the top euphotic zone. Still these benchtop flow cytometers provide reliable data for other populations such as heterotrophic bacteria and photosynthetic eukaryotes. Our study highlight the need for careful comparison between instruments before using them for large scale oceanographic surveys, using as reference the most sensitive laboratory instruments available. Supporting Information Additional Supporting Information may be found in the online version of this article. Filename Description lom310135-sup-0001-suppinfo.txt7.7 KB Supporting Information lom310135-sup-0002-suppinfoFigS1.tif20.9 MB Supporting Information Figure S1 lom310135-sup-0003-suppinfoFigS2.docx16.6 KB Supporting Information Figure S2 lom310135-sup-0004-suppinfoFigS3.tif9.6 MB Supporting Information Figure S3 Please note: The publisher is not responsible for the content or functionality of any supporting information supplied by the authors. Any queries (other than missing content) should be directed to the corresponding author for the article. References Bouvier, T., P. A. del Giorgio, and J. M. Gasol. 2007. A comparative study of the cytometric characteristics of High and Low nucleic-acid bacterioplankton cells from different aquatic ecosystems. Environ. Microbiol. 9: 2050– 2066. doi:10.1111/j.1462-2920.2007.01321.x Castro, B. M., F. P. Brandini, A. M. S. Pires-Vanin, and L. B. Miranda. 2006. Physical oceanography of the western Atlantic continental shelf located between 4 N and 34 S, p. 209– 251. In A. R. Robinson and K. H. Brink [eds.], The sea, volume 14A: The global coastal ocean. Harvard Univ. Press. Chisholm, S. W., R. J. Olson, E. R. Zettler, R. Goericke, J. B. Waterbury, and N. A. Welschmeyer. 1988. A novel free-living prochlorophyte abundant in the oceanic euphotic zone. Nature 334: 340– 343. doi:10.1038/334340a0 Crosbie, N., and M. Furnas. 2001. Abundance, distribution and flow- cytometric characterization of picophytoprokaryote populations in central (17oS) and southern (20oS) shelf waters of the Great Barrier Reef. J. Plankton Res. 23: 809– 828. doi:809-828. doi:10.1093/plankt/23.8.809 Dusenberry, J. A., and S. L. Frankel. 1994. Increasing the sensitivity of a FACScan flow cytometer to study oceanic picoplankton. Limnol. Oceanogr. 39: 206– 209. doi:10.4319/lo.1994.39.1.0206 Dusenberry, J. A., R. J. Olson, and S. W. Chisholm. 2001. Photoacclimation kinetics of single-cell fluorescence in laboratory and field populations of Prochlorococcus. Deep. Res. Part I Oceanogr. Res. Pap. 48: 1443– 1458. doi:10.1016/S0967-0637(00)00096-0 Gasol, J. M., and P. A. del Giorgio. 2000. Using flow cytometry for counting natural planktonic bacteria and understanding the structure of planktonic bacterial communities. Sci. Mar. 64: 197– 224. doi:10.3989/scimar.2000.64n2197 Kulk, G., W. H. van de Poll, R. J. W. Visser, and A. G. J. Buma. 2011. Distinct differences in photoacclimation potential between prokaryotic and eukaryotic oceanic phytoplankton. J. Exp. Mar. Bio. Ecol. 398: 63– 72. doi:10.1016/j.jembe.2010.12.011 Legendre, L., C. Courties, and M. Troussellier. 2001. Flow cytometry in oceanography 1989-1999: Environmental challenges and research trends. Cytometry 44: 164– 172. doi:10.1002/1097-0320(20010701)44:3 < 164::AID-CYTO1108 > 3.0.CO;2-6 Li, W. K. W. 1994. Primary production of prochlorophytes, cyanobacteria, and eucaryotic ultraphytoplankton: Measurements from flow cytometric sorting. Limnol. Oceanogr. 39: 169– 175. doi:10.4319/lo.1994.39.1.0169 Marie, D., F. Partensky, S. Jacquet, and D. Vaulot. 1997. Enumeration and cell cycle analysis of natural populations of marine picoplankton by flow cytometry using the nucleic acid stain SYBR Green I. Appl. Environ. Microbiol. 63: 186– 193. Marie, D., N. Simon, and D. Vaulot. 2005. Phytoplankton cell counting by flow cytometry, p. 253– 556. In R. A. Andersen [ed.], Algal culturing techniques. Academic Press. Morán, X., A. Bode, L. Suárez, and E. Nogueira. 2007. Assessing the relevance of nucleic acid content as an indicator of marine bacterial activity. Aquat. Microb. Ecol. 46: 141– 152. doi:10.3354/ame046141 Olson, R. J., D. Vaulot, and S. W. Chisholm. 1985. Marine phytoplankton distributions measured using shipboard flow cytometry. Deep Sea Res. Part A Oceanogr. Res. Pap. 32: 1273– 1280. doi:10.1016/0198-0149(85)90009-3 Olson, R. J., S. W. Chisholm, and E. R. Zettler. 1990. Pigments, size, and distribution of Synechococcus in the North Atlantic and Pacific Oceans. Limnol. Oceanogr. 35: 45– 58. doi:10.4319/lo.1990.35.1.0045 Ortega-Retuerta, E., I. Reche, E. Pulido-Villena, S. Agustí, and C. Duarte. 2008. Exploring the relationship between active bacterioplankton and phytoplankton in the Southern Ocean. Aquat. Microb. Ecol. 52: 99– 106. doi:10.3354/ame01216 Partensky, F., N. K. W. Hoepffner, O. Ulloa, and D. Vaulot. 1993. Photoacclimation of Prochlorococcus sp. (Prochlorophyta) Strains isolated from the northern Atlantic and the Mediterranean sea. Plant Physiol. 101: 285– 296. Partensky, F., J. Blanchot, and D. Vaulot. 1999a. Differential distribution and ecology of Prochlorococcus and synechococcus in oceanic waters: A review. Bull. l'Institut océanographique. Partensky, F., W. R. Hess, and D. Vaulot. 1999b. Prochlorococcus, a marine photosynthetic prokaryote of global significance. Microbiol. Mol. Biol. Rev. 63: 106– 127. Scanlan, D. J., and others. 2009. Ecological genomics of marine picocyanobacteria. Microbiol. Mol. Biol. Rev. 73: 249– 299. doi:10.1128/MMBR.00035-08 Shapiro, H. M. 2003. Practical flow cytometry, 3rd ed. In H. M. Shapiro, M.D., Wiley-Liss, Inc., 1995, 4th ed. 542 p. Simon, N., R. G. Barlow, D. Marie, F. Partensky, and D. Vaulot. 1994. Characterization of oceanic photosynthetic picoeukaryotes by flow-cytometry. J. Phycol. 30: 922– 935. doi:10.1111/j.0022-3646.1994.00922.x Sosik, H. M., S. W. Chisholm, and R. J. Olson. 1989. Chlorophyll fluorescence from single cells: Interpretation of flow cytometric signals. Limnol. Oceanogr. 34: 1749– 1761. doi:10.4319/lo.1989.34.8.1749 Team R Development Core 2013. R: A language and environment for statistical computing, p. 1– 3464. R Found. Stat. Comput. Thompson, A. W., and G. van den Engh. 2016. A multi-laser flow cytometry method to measure single cell and population-level relative fluorescence action spectra for the targeted study and isolation of phytoplankton in complex assemblages. Limnol. Oceanogr. Methods 14: 39– 49. doi:10.1002/lom3.10068 Trask, B. J., G. J. van den Engh, and J. H. Elgershuizen. 1982. Analysis of phytoplankton by flow cytometry. Cytometry 2: 258– 264. doi:10.1002/cyto.990020410 Van Wambeke, F., P. Catala, M. Pujo-Pay, and P. Lebaron. 2011. Vertical and longitudinal gradients in HNA-LNA cell abundances and cytometric characteristics in the Mediterranean Sea. Biogeosciences 8: 1853– 1863. doi:10.5194/bg-8-1853-2011 Vaulot, D., C. Courties, and F. Partensky. 1989. A simple method to preserve oceanic phytoplankton for flow cytometric analyses. Cytometry 10: 629– 635. doi:10.1002/cyto.990100519 Vaulot, D., and D. Marie. 1999. Diel variability of photosynthetic picoplankton in the equatorial Pacific. J. Geophys. Res. Oceans 104: 3297– 3310. doi:10.1029/98JC01333 Vives-Rego, J., P. Lebaron, and G. Nebe-von Caron. 2000. Current and future applications of flow cytometry in aquatic microbiology. FEMS Microbiol. Rev. 24: 429– 448. doi:10.1111/j.1574-6976.2000.tb00549.x Zubkov, M. V., M. A. Sleigh, G. A. Tarran, P. H. Burkill, and R. J. Leakey. 1998. Picoplanktonic community structure on an Atlantic transect from 50°N to 50°S. Deep Sea Res. Part I Oceanogr. Res. Pap. 45: 1339– 1355. doi:10.1016/S0967-0637(98)00015-6 Acknowledgments We thank the officers and crew of the R/V Alpha Crucis for logistical support. Financial support for this work was provided by the CNRS Groupement de Recherche International (GDRI) “Diversity, Evolution and Biotechnology of Marine Algae”, the COFECUB-CAPES project “Pico Brás” (TE 871-15) and FAPESP - Fundação de Amparo à Pesquisa do Estado de São Paulo (2012/04800-9 and 2014/15242-2). Citing Literature Volume14, Issue11November 2016Pages 750-758 FiguresReferencesRelatedInformation
Referência(s)