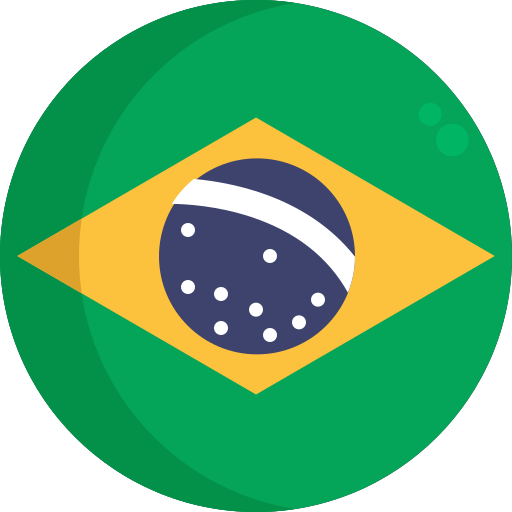
Minimising direct‐coupled distributed synchronous generators impact on electric power systems protection
2019; Institution of Engineering and Technology; Volume: 13; Issue: 18 Linguagem: Inglês
10.1049/iet-gtd.2018.6757
ISSN1751-8695
AutoresFernando Guilherme Kaehler Guarda, Ghendy Cardoso, Ubiratan Holanda Bezerra, João Paulo Abreu Vieira,
Tópico(s)HVDC Systems and Fault Protection
ResumoIET Generation, Transmission & DistributionVolume 13, Issue 18 p. 4190-4196 Research ArticleFree Access Minimising direct-coupled distributed synchronous generators impact on electric power systems protection Fernando Guilherme Kaehler Guarda, Corresponding Author Fernando Guilherme Kaehler Guarda fernandokg@gmail.com orcid.org/0000-0001-7957-9462 Centro de Excelência em Energia e Sistemas de Potência, Universidade Federal de Santa Maria, Av. Roraima, 1000, Santa Maria, BrazilSearch for more papers by this authorGhendy Cardoso Jr., Ghendy Cardoso Jr. Centro de Excelência em Energia e Sistemas de Potência, Universidade Federal de Santa Maria, Av. Roraima, 1000, Santa Maria, BrazilSearch for more papers by this authorUbiratan Holanda Bezerra, Ubiratan Holanda Bezerra Centro de Excelência em Energia e Sistemas de Potência, Universidade Federal de Santa Maria, Av. Roraima, 1000, Santa Maria, BrazilSearch for more papers by this authorJoão Paulo Abreu Vieira, João Paulo Abreu Vieira Technological Institute - ITEC, Universidade Federal do Pará, Rua Augusto Corrêa, 1, Belém, BrazilSearch for more papers by this author Fernando Guilherme Kaehler Guarda, Corresponding Author Fernando Guilherme Kaehler Guarda fernandokg@gmail.com orcid.org/0000-0001-7957-9462 Centro de Excelência em Energia e Sistemas de Potência, Universidade Federal de Santa Maria, Av. Roraima, 1000, Santa Maria, BrazilSearch for more papers by this authorGhendy Cardoso Jr., Ghendy Cardoso Jr. Centro de Excelência em Energia e Sistemas de Potência, Universidade Federal de Santa Maria, Av. Roraima, 1000, Santa Maria, BrazilSearch for more papers by this authorUbiratan Holanda Bezerra, Ubiratan Holanda Bezerra Centro de Excelência em Energia e Sistemas de Potência, Universidade Federal de Santa Maria, Av. Roraima, 1000, Santa Maria, BrazilSearch for more papers by this authorJoão Paulo Abreu Vieira, João Paulo Abreu Vieira Technological Institute - ITEC, Universidade Federal do Pará, Rua Augusto Corrêa, 1, Belém, BrazilSearch for more papers by this author First published: 04 September 2019 https://doi.org/10.1049/iet-gtd.2018.6757Citations: 2AboutSectionsPDF ToolsRequest permissionExport citationAdd to favoritesTrack citation ShareShare Give accessShare full text accessShare full-text accessPlease review our Terms and Conditions of Use and check box below to share full-text version of article.I have read and accept the Wiley Online Library Terms and Conditions of UseShareable LinkUse the link below to share a full-text version of this article with your friends and colleagues. Learn more.Copy URL Share a linkShare onFacebookTwitterLinkedInRedditWechat Abstract This study presents a method to place, size, coordinate and adequate protection systems in distribution networks (DNs) with directly-coupled distributed generation (DG). DG penetration increase in DNs is beneficial to the operation of these grids. However, since the majority of DN is already consolidated, with protective devices sized for certain current levels and directionality, adding DG to these systems may cause issues. This study introduces a method to determine protective devices placement, their coordination and sizing, depending on DG location. Direct-coupled DG is considered since DG connected to DNs through power electronics limit the fault current to the network. Firstly, recloser allocation is treated in a multiobjective approach, reducing reliability indices. To solve this problem, particle swarm optimization is applied. Fuse cutouts allocation is determined by a set of rules, developed considering feeder particularities. After, DG is considered and its effects on the protection coordination are evaluated. To adapt DN to receive DG, fault current limiters are placed to avoid recloser – fuse miscoordination. Finally, recloser operation is analyzed to determine the operational philosophy to avoid misoperation in the presence of DG. Graphical and numerical results are presented for test systems to show the functionality and performance of the proposed method. 1 Introduction The operation of traditional electric distribution systems (EDS) is changing due to the development of new technologies that aim to improve energy supply. With smart grids in evidence, also grows distributed generation (DG) penetration in EDS. This distribution sources can reduce power losses in distribution systems, improve energy quality and voltage profile, among others [1]. However, the increase in DG penetration in traditional EDS causes problems that must be addressed, such as voltage control difficulties, reactive energy management problems, unintentional islanding and protective devices miscoordination, among others [2]. Distribution systems have their protective devices allocated before considering DG penetration, having their coordination already established. DG contribution for fault current alters the protective devices sensitivity, causing several miscoordination problems, demanding new protection studies [3]. In order to solve miscoordination problems, several authors developed methods to reduce the influence of DG on protective devices coordination. In [4], an adaptive overcurrent protection of distribution network (DN) with DG is presented. The method considers islanding detection, grid re-synchronisation and characteristics of downstream relays as an input, measuring voltage, current and frequency. However, the method must calculate the time taken to eliminate the fault in order to change the settings of relays, so it will allow the fault to last. Moreover, fuse cutouts and reclosers are not contemplated, which makes the method not able for real application. Kumar et al. [5] claim to have developed an adaptive relay mechanism for the complete protection of DN with DG penetration. Although the Fourier algorithm for tracking power system signals was proved efficient, the method is not applicable to the complete protection of DN, since the authors considered the protection system composed only by overcurrent relays. Mahat et al. [4] developed a multiagent system-based protection and control scheme to deal with several operational conditions in DN with DG. The method is based on the cooperation between DG controller and relays, being developed an adaptive protection and control algorithm designed on a converter-based wind turbine DG to limit the influence of infeed fault current. With this method, the relays have their settings adapted, considering DG control modes. The method considers only relay settings, disregarding recloser settings, which are essential in DN to avoid unnecessary interruptions. In [6], the authors claim to have solved recloser–fuse miscoordination in DN with DG. The method consists in place fault current limiter (FCL) to reduce fault current to the coordination zone determined by recloser and fuse time–current characteristics. However, this method does not take into account the possibility of DG feeding the fault during recloser fast operation, which makes their practical use unfeasible. In this paper, direct-coupled DG is considered since distributed sources connected to DNs through power electronics can limit the fault current to the network. This paper aims to provide a solution for the worst case, which is when a direct-coupled DG contributes to the fault. Hence, a method is presented to allocate protection devices and FCL in order to maintain recloser–fuse coordination and fuse selectivity in DN with direct-coupled DG. The allocation of protective devices is divided into two steps: firstly, fuse cutouts placement is carried out following a set of rules developed considering the characteristic of feeders, such as importance of loads, physical location and section length, among others. In a second moment, recloser placement is determined considering a multiobjective approach, aiming to improve reliability indices. The optimisation method is modelled by a multiobjective weighted particle swarm optimisation (PSO). After determining protective devices placement, DG is introduced in the DN. FCL placement is then carried out, aiming to reduce current differences between fuses and also reducing the number of FCL to be installed. Moreover, recloser operational philosophy is determined, in order to avoid DG infeed during recloser fast operation. The method is tested in two DN. The authors consider the following contributions to be original: An actual solution for mitigating direct-coupled DG influence on the EDS protection is presented, considering not only fault magnitude, but also coordination and selectivity aspects between protective devices; FCL optimal placement is solved using a multiobjective approach, which maintains selectivity between fuse cutouts, thus reducing selectivity violations; Recloser philosophy (coordinated – fuse saving or selective – fuse blowing) is modified depending on DG location; Fuse cutout selectivity is assured when DG is present or not, disconnecting the minimum number of consumers 2 Problem statement 2.1 DG influence in electric distribution system protection Fig. 1 shows a traditional radial distribution feeder that consists in main feeder and branches that supply loads. The protection system is composed by a substation overcurrent relay, recloser and fuse cutouts protecting lateral branches that feed distribution transformers. Fig. 1Open in figure viewerPowerPoint Traditional radial distribution system configuration With the insertion of DG sources (directly coupled synchronous generators), the fault current will be the sum of substation contribution and DG contribution for the fault, as shown in Fig. 2. Fig. 2Open in figure viewerPowerPoint Substation and DG contribution for fault current Current methodologies state that allocating a FCL to limit DG contribution will solve recloser–fuse miscoordination [7–9], which is a mistake. Considering a coordinated protection system, when a temporary fault occurs, recloser will operate in its fast curve. However, depending on DG location, there still will be contribution from DG sources downstream of the fault, and it may cause fuse cutout to blow or the recloser fast curve operation will not clear the fault since the dielectric characteristic of the air will not recover, regardless of nature of the fault (temporary or permanent), as shown in Fig. 3. Therefore, depending on DG short circuit power, it will be impossible to eliminate the electric arc during recloser fast operation, which invalidates these methodologies. Fig. 3Open in figure viewerPowerPoint Fuse blowout due to DG contribution for the fault current In order to avoid recloser fast operation and fuse blowout due to DG contribution, it is imperative that recloser operational philosophy is changed from coordinated to selective, and that this extra contribution for fault current by DG does not affect fuse cutout selectivity. Hence, to solve this problem, this paper will present a methodology to allocate FCL to reduce the influence of DG on recloser–fuse miscoordination as well as establish recloser operation philosophy (coordinated or selective) in order to maintain an acceptable reliability level. 2.2 Particle swarm optimisation PSO algorithm is a stochastic search method inspired by the coordinated movement of groups of animals. The change in direction and speed of each individual particle results from the effect of cognitive, social and stochastic influences. The common goal of all particles is to find the most favourable location within a search space. PSO can be used to solve problems in the form (1)s.t. (2) (3) (4) (5) (6)where , , , and are vectors, and represents the lower bound and the upper bound. and are matrices. The functions , and can be non-linear. The fitness function quantifies the performance of . This work adopts a formulation for PSO as shown in (7) and (8), where particles speed and position are updated, respectively (7) (8)In this formulation, and are vectors that represent the position and velocity of particle i, on the kthgeneration; is the best personal position of each individual; G is the best global position observed between all the particles in the swarm, until the current generation; and are uniformly distributed values between [0,1]; and are acceleration constants; is inertia function. The PSO graphic representation is shown in Fig. 4, in a bi-dimensional search space. The new velocity, , is the sum of the momentum that tends to maintain the particle in its current course. This particle is attracted to its personal best P and finally, there is an attraction of the whole swarm towards the global best G. The new position, , is the sum of the current position and the velocity . Fig. 4Open in figure viewerPowerPoint PSO graphical representation 2.2.1 Objective functions Firstly, a short circuit calculation is performed in all system nodes. This preliminary calculation is a reference to be used in the optimisation process. Hence, the number, placement and specification of DG that can be connected to any system node is made, allowing any combination of DG. Considering all DG connected, a new short circuit calculation is made and thus the new fault currents are determined. The objective functions modelled for FCL allocation problem aim to minimise the difference between fault currents that the protective devices are subject to without considering the installation of DG sources and after the connection of DG. Also, the size of the FCL must be minimised, thus restricting the associated cost. The objective functions are shown as follows: (9) (10)where is the number of protecting fuse cutouts; is the fault current on the nth protecting fuse cutout, considering DG penetration; is the fault current on the nth protecting fuse cutout, without DG penetration; is the number of protected fuse cutouts; is the fault current on the pth protected fuse, considering DG penetration; is the fault current on the pth protected fuse, without DG penetration; is the number of FCLs; is the resistance of the kth FCL; is the inductance of the kth FCL. These objective functions aim to determine FCL placement in order to reduce fault current difference between protecting and protected fuse cutouts with and without DG, in order to maintain selectivity. Also, FCL size must be minimised, to reduce costs, since these devices costs depend on their impedances. 2.2.2 Problem restrictions Restrictions must reflect technical and economical boundaries, in order to adequate the solution to a feasible region on the search space (11) (12) (13) (14)where is the current on the ith feeder section, considering DG; is the current on the ith feeder section, before DG connection; Tol is the tolerance for the difference between currents before and after DG connection; and are the FCL reactance limits ; and are the FCL resistance limits ; is the selective fault current in protecting fuse cutout k; is the selective fault current in protected fuse cutout k; is the selectivity current limit between and . Restriction (11) states that the fault current difference in feeder section i with and without DG contribution must be lower than a pre-established tolerance. Restrictions (12) and (13) limit FCL size between a minimum and maximum value of reactance and resistance, respectively. Restriction (14) relates to current selectivity limits between fuse cutouts, which must be met. 2.2.3 Solution method: multiobjective PSO To solve multi-objective problems, the Pareto solutions (non-dominated) must be maximised. In multiobjective PSO, it is necessary to decide how to select the particles to give preference to those non-dominated and how to maintain the diversity of the swarm. The implementation of multiobjective PSO algorithm is shown in the flowchart of Fig. 5. Fig. 5Open in figure viewerPowerPoint Multiobjective PSO 2.3 Recloser operational philosophy To determine if recloser fast characteristic should be on (coordinated) or off (selective), DG position must be investigated. Previously, researchers found in the literature do not consider DG position, and that is proven to be a mistake because of DG contribution to temporary faults when recloser opens the circuit in its fast characteristic. When DG is located downstream of the recloser, and a fault occurs between these two, there will be a fuse blowout due to DG contribution, making fuse saving scheme not suitable, because there will not be enough time to eliminate the electric arc. To solve this problem, recloser operation philosophy should be switched from coordinated to selective, disabling recloser fast characteristic and allowing the fuse cutout to blow, disconnecting the minimum number of consumers. Fig. 6 shows a different situation, where DG is located upstream the recloser. This configuration will significantly increase fault current for faults downstream of the recloser and cause recloser–fuse miscoordination, although it will not require recloser philosophy alteration. This paper solved this situation through the FCL allocation. Fig. 6Open in figure viewerPowerPoint Substation and DG contribution for DG source located upstream from recloser Also in Fig. 6 it is possible to notice that when a DG source is located downstream from the recloser, there will be an extra contribution for a fault, thus, damaging recloser–fuse coordination. 3 Test results In order to evaluate the method's efficiency against recloser–fuse miscoordination, it is considered a test system widely used in the literature. The considered radial test system is shown in Fig. 7, and it was chosen because of the available data [10], being modelled in Matlab for mathematical purposes and Digsilent® to evaluate technical characteristics. Fig. 7Open in figure viewerPowerPoint Test system – IEEE 37 nodes Protective devices placement is shown in Table 1. Three DG sources, consisting in synchronous generators, are considered separately and combined, connected to the network directly through a transformer and all combination of DG connections are addressed. DG data is found in Table 2. Recloser and fuse cutouts are previously sized and coordinated, using a traditional method [11]. Table 3 shows FCL allocation and sizing and the adequate recloser operational philosophy for the considered test systems. Faults were simulated in nodes 728, 740 and 775, which represent three different fault directions regarding recloser's positions. In Table 4, the results are presented for the worst-case scenario, depending on which DG is connected. Table 1. Protective devices placement sections IEEE 37 nodes OC relay Reclosers Fuse cutouts 799-701 702-703 (Pos. 1); 708-733 (Pos. 2) 702-705; 702-713; 704-720; 703-727; 709-731; 709-775; 708-732; 734-710; 711-740 Table 2. DG sources data Parameter Data power 2 MVA synchronous reactance (Xs) 2.95% transient reactance (X′d) 0.25% sub-transient reactance (X″d) 0.17% direct-axis transient short-circuit time constant (T′d) 0.47 direct-axis sub-transient short-circuit time constant (T″d) 0.054 DG reactance 9.67% DG transformer 5%, 12.47 kV/480 V base voltage 12.47 kV base MVA 100 MVA Table 3. FCL placement, sizing and recloser philosophy for IEEE 37 nodes DG combination FCL locations FCL sizes DG1 DG1; 730-709 j0.48; 0.48 + j0.32 DG2 DG2; 730-709 0.1 + j0.28; 0.48 + j0.32 DG3 DG3; 730-709 0.3 + j0.36; 0.48 + j0.32 DG1 + DG2 DG1; DG2; 730-709; 704-713 j0.48; 0.1 + j0.28; 0.48 + j0.32; 0.15 + j0.22 DG2 + DG3 DG2; DG3; 730-709; 704-713; 727-744 0.1 + j0.28; 0.3 + j0.36; 0.48 + j0.32; 0.15 + j0.22 DG1 + DG3 DG1; DG3; 727-744; 730-709; j0.48; 0.3 + j0.36; 0.48 + j0.32; 0.15 + j0.22 DG1 + DG2 + DG3 DG1; DG2; DG3; 704-713; 730-709; 727-744 j0.48; 0.1 + j0.28; 0.3 + j0.36; 0.48 + j0.32; 0.15 + j0.22 Table 4. Protection operating times with DG For faulted node 775 and DG 3 R1 40 K fuse DG3 protection time gap 0.08 0.35 0.2 0.12 For faulted node 775 and DG 3 R2 15 K fuse DG1 protection time gap 0.12 0.25 0.2 0.08 The method developed in [6] was implemented in order to assess the effectiveness of the proposed method, considering the same DG, faulted nodes and protective devices. It is possible to see that, in case 1, there will be the fast operation of the recloser for a fault in 728. However, DG will continue to contribute for the fault, preventing reclosing and even causing fuse cutout to blow. Recloser philosophy in this case must be selective in order to disconnect only consumers downstream of 728. In case 2, the coordinated recloser philosophy can be applied due to the fault current sensed by the recloser being composed by the sum of substation and DG. This temporary fault will be removed by recloser's fast operation. In Fig. 8 it is possible to notice that recloser fast operation is faster than fuse cutout, which means that coordination is successfully achieved. What Hussain et al. [6] do not consider is DG contribution during recloser fast operation, when DG is located upstream from the recloser. For higher current values, considering DG contribution, coordination is lost due to this extra contribution for the fault. The proposed method proposes the application of FCLs to fix this problem. Protection times are shown in Table 4. Fig. 8Open in figure viewerPowerPoint Operating time from devices in [6] It is clear that a time gap between DG protection and recloser fast operation is present. During this time gap, DG remains contributing for the fault, which can cause fuse cutout operation. Fig. 9 shows the protective devices operating devices obtained by implementing the method developed in [6], considering DG. Fig. 9Open in figure viewerPowerPoint Operating time from devices in [6] with DG The developed method successfully corrected the problem of DG feeding the fault during recloser fast operation by changing its philosophy from coordinated to selective, as shown in Table 5. Through this philosophy modification, protection system becomes selective, where the fuse cutout is the first responsible to clear the fault, avoiding DG contribution for the fault. Table 5. Recloser philosophy for different faults Case DG combination Faulted node (728) Faulted node (740) Faulted node (775) 1 DG1 R1: S R1: C R1: C R2: C R2: S R2: S 2 DG2 R1: C R1: C R1: C R2: C R2: C R2: C 3 DG3 R1: S R1: S R1: C R2: C R2: C R2: C 4 DG1 + DG2 R1: S R1: C R1: S R2: C R2: S R2: C 5 DG2 + DG3 R1: S R1: C R1: S R2: C R2: C R2: C 6 DG1 + DG3 R1: S R1: C R1: S R2: C R2: S R2: C 7 DG1 + DG2 + DG3 R1: S R1: C R1: S R2: S R2: S R2: S 4 Conclusions and recommendations The developed methodology was able to efficiently allocate FCLs in EDS in order to reduce selectivity violations between protective devices. Also, unlike several previously developed methods, this technique successfully considered recloser–fuse miscoordination, taking into account recloser operational philosophy selection (fuse saving or not). Comparing the developed method to a method previously proposed in the literature, it became clear that the problem of DG feeding the fault during recloser fast operation is an issue that the proposed method has successfully solved. FCL placement is responsible for limiting DG contribution to fault current, in order to avoid selectivity violations between fuse cutouts and between fuse links and reclosers, in appropriate situations. The method was applied in a widely used test system for evaluation. The results showed excellent performance, successfully avoiding selectivity violations between fuse cutouts and, consequently, between fuse cutouts and reclosers. Also, reclosers’ operational philosophy was changed depending on DG location, avoiding fuse cutouts to blow or failure of reclosing attempts while DG continues to feed a temporary fault during the reclosing interval after recloser fast operation. Also, it was possible to notice that when distribution systems receive DG, fuse saving philosophy is not suitable due to DG contribution for the fault. 5 Acknowledgments This work was developed under support of CEA – the electric utility of the State of Amapá – Brazil, through a R&D project executed by Federal University of Santa Maria, and Federal University of Pará – Brazil. The authors thank the Coordination for the Improvement of High Level Personnel (CAPES). 6 References 1Ochoa, L.F., Feltrin, A.P., Harrison, G.P.: ‘Evaluating distributed generation impact with a multiobjective index’, IEEE Trans. Power Deliv., 2006, 21, (3), pp. 1452– 1458 2Manditereza, P.T., Bansal, R.: ‘Renewable distributed generation: the hidden challenges – a review from the protection perspective’, Renew. Sust. Energy Rev., 2016, 58, pp. 1457– 1465 3Kennedy, J., Ciufo, P., Agalgaonkar, A.: ‘A review of protection systems for distribution networks embedded with renewable generation’, Renew. Sust. Energy Rev., 2016, 58, pp. 1308– 1317 4Mahat, P., Chen, Z., Bak-Jensen, B., et al.: ‘A simple adaptive overcurrent protection of distribution systems with distribution generation’, IEEE Trans. Smart Grid, 2011, 2, (3), pp. 428– 437 5Kumar, D.S., Srinivasan, D., Reindl, T.: ‘A fast and scalable protection scheme for distribution networks with distributed generation’, IEEE Trans. Smart Grid, 2016, 31, (1), pp. 67– 75 6Hussain, B., Sharkh, S.M., Hussain, S., et al.: ‘An adaptive relaying scheme for fuse saving in distribution networks with distributed generation’, IEEE Trans. Power Deliv., 2013, 28, (2), pp. 669– 677 7Hussain, B., Sharkh, S.M., Hussain, S., et al.: ‘An adaptive relaying scheme for fuse saving in distribution networks with distribution generation’, IEEE Trans. Power Deliv., 2013, 28, (2), pp. 669– 677 8Shah, P.H., Bhalja, B.R.: ‘New adaptive digital relaying scheme to tackle recloser – fuse miscoordination during distributed generation interconnections’, IET Gener. Transm. Distrib., 2014, 8, (4), pp. 682– 688 9Chaitusaney, S., Yokoyama, A.: ‘Prevention of reliability degradation from recloser – fuse miscoordination due to distributed generation’, IEEE Trans. Power Deliv., 2008, 23, (4), pp. 2545– 2554 10 IEEE distribution planning working group report.: ‘Radial distribution test feeders’, IEEE Trans. Power Syst., 1991, 6, (3), pp. 975– 985 11Gönen, T.: ‘ Electric power distribution engineering’ ( CRC Press, New York, NY, USA, 2014, 3rd edn.) Citing Literature Volume13, Issue18September 2019Pages 4190-4196 FiguresReferencesRelatedInformation
Referência(s)