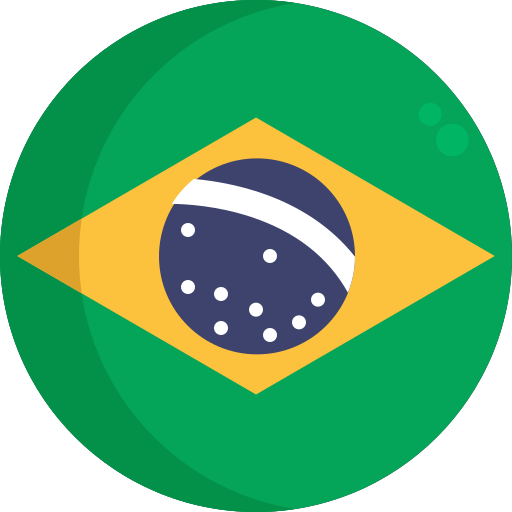
Analysis of alternative parameters of dynamic resistance measurement in high voltage circuit breakers
2019; Institution of Engineering and Technology; Volume: 4; Issue: 3 Linguagem: Inglês
10.1049/hve.2019.0046
ISSN2096-9813
AutoresHerbet Filipe dos Santos Sousa, A. Oliveira, George Victor Rocha Xavier, Henrique Nunes de Santana, Edson Guedes da Costa, Tarso Vilela Ferreira,
Tópico(s)Electrical Fault Detection and Protection
ResumoHigh VoltageVolume 4, Issue 3 p. 197-202 Special Issue: Selected Papers from the 2018 IEEE International Conference on High Voltage Engineering and Application (ICHVE 2018)Open Access Analysis of alternative parameters of dynamic resistance measurement in high voltage circuit breakers Herbet Filipe dos Santos Sousa, Corresponding Author Herbet Filipe dos Santos Sousa herbet.sousa@ee.ufcg.edu.br Federal University of Campina Grande, Campina Grande, BrazilSearch for more papers by this authorAdriano Costa de Oliveira, Adriano Costa de Oliveira Federal University of Campina Grande, Campina Grande, BrazilSearch for more papers by this authorGeorge Victor Rocha Xavier, George Victor Rocha Xavier Federal University of Campina Grande, Campina Grande, BrazilSearch for more papers by this authorHenrique Nunes de Santana, Henrique Nunes de Santana Federal University of Campina Grande, Campina Grande, BrazilSearch for more papers by this authorEdson Guedes da Costa, Edson Guedes da Costa Federal University of Campina Grande, Campina Grande, BrazilSearch for more papers by this authorTarso Vilela Ferreira, Tarso Vilela Ferreira Federal University of Sergipe, Aracaju, BrazilSearch for more papers by this author Herbet Filipe dos Santos Sousa, Corresponding Author Herbet Filipe dos Santos Sousa herbet.sousa@ee.ufcg.edu.br Federal University of Campina Grande, Campina Grande, BrazilSearch for more papers by this authorAdriano Costa de Oliveira, Adriano Costa de Oliveira Federal University of Campina Grande, Campina Grande, BrazilSearch for more papers by this authorGeorge Victor Rocha Xavier, George Victor Rocha Xavier Federal University of Campina Grande, Campina Grande, BrazilSearch for more papers by this authorHenrique Nunes de Santana, Henrique Nunes de Santana Federal University of Campina Grande, Campina Grande, BrazilSearch for more papers by this authorEdson Guedes da Costa, Edson Guedes da Costa Federal University of Campina Grande, Campina Grande, BrazilSearch for more papers by this authorTarso Vilela Ferreira, Tarso Vilela Ferreira Federal University of Sergipe, Aracaju, BrazilSearch for more papers by this author First published: 19 September 2019 https://doi.org/10.1049/hve.2019.0046Citations: 7AboutSectionsPDF ToolsRequest permissionExport citationAdd to favoritesTrack citation ShareShare Give accessShare full text accessShare full-text accessPlease review our Terms and Conditions of Use and check box below to share full-text version of article.I have read and accept the Wiley Online Library Terms and Conditions of UseShareable LinkUse the link below to share a full-text version of this article with your friends and colleagues. Learn more.Copy URL Share a linkShare onFacebookTwitterLinkedInRedditWechat Abstract Throughout its lifetime, high-voltage circuit breaker (HVCB) contacts suffer from inevitable degradation processes, especially during the formation of electric arcs. Highly degraded contacts can reduce the current interruption capacity and increase the chances of operational faults, which might lead to considerable financial losses. Therefore, the use of efficient evaluating techniques is a key factor to achieve systems reliability. The dynamic resistance measurement (DRM) is a technique that can be used to evaluate the degradation level of HVCB' contacts. It stands out from the usual technique, static resistance measurement, by allowing the assessment of both the main contacts and the arcing contacts (ACs). Usually, the mean resistance or the area under the DRM curve is used to estimate the AC degradation. However, DRM is a non-standardised technique and there are no specified parameters to evaluate the real wear conditions. Therefore, an analysis of alternative parameters extractible from the DRM technique is presented in this study, such as amplitude and variance of the DRM curve. The results attest the possible use of more than one parameter for the continuous evaluation of HVCB ACs, characterising the DRM as a more complete and precise diagnosis tool. 1 Introduction High-voltage circuit breakers (HVCBs) are electromechanical equipment capable of establishing and interrupting the flow of electric current in power circuits [1]. In addition, when designed to operate, this equipment must be capable of extinguishing the electric arc effectively, which is caused by the switching of high voltage circuits. The degradation of the mechanical and electrical components of HVCBs is an inevitable and continuous process, due to the accumulated efforts in these items along its usage. Given the importance of circuit breakers in the electrical system, researchers have contributed over time to the advancement of monitoring techniques for the circuit breaker operating status since 1940 [2]. In monitoring techniques on HVCB, the mechanical aspects, the control circuits and the arc extinguishing chamber are commonly evaluated. The evaluation of the other parts of the circuit-breaker is not such a complicated task, compared to the evaluation of the electric arc extinguishing chamber [3]. The reason for this is that this part of the circuit breaker is encapsulated and difficult to access. The electric arc extinguishing chamber plays a fundamental role in the operation of the circuit breaker and is responsible for the main functions of the equipment. The processes of opening and closing the circuit in the circuit breaker are performed by the fixed and mobile contact pairs present inside the extinguishing chamber. Each contact (fixed or mobile) has two distinct contact regions [main contact (MC) and arcing contact (AC)]. The contact degradation is mainly related to the electric arc and mechanical stresses generated during the HVCB operation. This degradation can result in contact shortening due to erosion, contact surface deformation and increase of the electrical resistance. As a consequence, the HVCB current interruption capacity can be compromised, affecting the reliability of the protection system and compromising the integrity of the system components. The main techniques to verify the degradation levels of the contacts are based on the measurement of electrical resistance [4]. Since the resistance of the contacts, which should be minimal, increases as they wear out, measurement of the electrical resistance of the contacts is carried out without dismantling the extinguishing chamber. Currently, the standard technique (ANSI C37.09 and IEC62271 1) and most commonly used among maintenance plans of electric utilities is static resistance measurement (SRM). However, SRM is limited to the MC evaluation, for which the measurement is carried out with the circuit breaker in the closed position (with the direct conduction of electric current). Therefore, information about the AC is neglected [5]. Dynamic resistance measurement (DRM) is then proposed to meet the need for methods that also evaluate AC [6]. This technique is based on the continuous measurement of the electrical resistance during the operation of the HVCB (also measuring the pre-establishment times of the electric arc), from which a resistance curve is acquired over time (or as a function of the mobile contact displacement) [7, 8]. In this way, DRM analysis provides a more detailed and precise evaluation of the degradation state of the contact, allowing the identification of critical degradation points from variations in the resistance curve pattern. The DRM tests must be performed with the circuit breaker out of operation. The test circuit should present a current injection system capable of delivering, at least, a DC of 50 or 100 A, according to ANSI C37.09 and IEC 62271-1 standards, respectively [9, 10]. However, DRM is currently not a consolidated technique. The absence of reference values by the manufacturers for the AC wear evaluation, together with the non-standardisation of effective evaluation parameters; contribute to the non-inclusion of this technique in the maintenance plans in electrical substations [11]. Although DRM is not a consolidated procedure, research works about the technique are conducted since 1995 [6], in which the contact degradation levels were defined according to the AC lengths. However, this parameter presented itself as ineffective, since the AC can reach high degradation levels without significant changes in their lengths for several circuit breakers models. The method proposed by Landry et al.[12] is highlighted by the contacts opening velocity reduction and the use of the area under the DRM curve as an evaluating parameter for contact degradation, presenting good success diagnosis rates. The opening speed reduction provides lower contact vibrations during measurements, resulting in a resistance curve with minor oscillations and, consequently, easier interpretation. In addition, the resistance curve obtained for a controlled speed presents a greater constancy in the area under the curve values, reinforcing the use of this parameter for the diagnosis of the contacts. The value of the area below the AC region in the DRM curve corresponds proportionally to the thermal energy associated with the process of contacts separation, which in turn is related to its wear state. Nonetheless, in [13] it is reported that the method presented by [12] can damage some breaker mechanism when restoring it back into service since it requires the substitution of the standard operating mechanism by an auxiliary mechanical device. However, in [14] it is presented that the DRM can be used at the nominal speed since the injection system has a value of 300 A as applied DC current. In addition, [14] reinforces the use of the area under the DRM curve as a valid evaluating parameter for contact degradation. Nonetheless, the exclusive use of the area below the curve value as an evaluating parameter may not be efficient in the diagnosis of some specific problems. In cases where the contacts have a punctual degradation, the resistance curve exhibits a resistance rise only at specific instants, which does not necessarily result in a significant increase in the area below the curve. In addition, the identification of the time instants for the main and arc contacts separation is not exact, which can significantly affect the area calculation. Therefore, for the complete consolidation of the DRM as contact degradation level technique, additional parameters beyond the area under the DRM curve should be addressed. Additional evaluating parameters were studied over the years, such as: the level of mass transferred by the contacts [15]; acoustic vibration [16]; average arcing and MC resistances (MCRs) [17, 18]; time difference between separation of the main and AC [19] and; maximum resistance over the entire curve [20]. Even so, for some DRM curves, the values of the mentioned evaluating parameters present similar values for low- and high-level degraded contacts, exposing the need of the inclusion of new evaluating parameters that can aid in the highlighting of the differences between contacts degradation levels. In this scenario, the present work proposes to evaluate new alternative parameters based on ACs amplitude (ACAm) and variance (ACV) of the DRM curve, for degradation analysis of HVCB AC, aiming to aid in the DRM technique consolidation process for contacts degradation diagnosis. For this purpose, five pairs of circuit breaker contacts with different levels of degradation were selected. Each of the contacts pairs was subjected to ten DRM tests, resulting in 50 DRM curves to be analysed. 2 Dynamic resistance measurement To perform the DRM in an HVCB installed in a substation, the first process is to withdraw the equipment, doing its matching insulation. Since the proper insulation of the energised parts is done, a measuring instrument (circuit breaker analyser) is installed for the DRM test between the circuit breaker terminals, as shown in Fig. 1. Fig. 1Open in figure viewer DRM execution scheme [9] The instrument provides a current source and measures voltage and current parameters during circuit breaker operation. With the values obtained, the dynamic electric resistance curve is calculated as a function of time or the displacement of the moving contact. The resistance curve acquired in the DRM test (performed at the breaker opening) results in four main regions of different meanings, as shown in Fig. 2. Fig. 2Open in figure viewer DRM curve sample for a test current of 300 A The blue region refers to the MCR, in which the current flows through the MCs. The resistance in the blue region matches the static resistance value (obtained with closed contacts). The red region is considered as a transition zone, since the main and ACs are connected in parallel, sharing the test current. Finally, the yellow region refers to the AC resistance (ACR), delimited by the end of the transition region until the total galvanic separation, represented by the green region, in which the resistance values increase abruptly, since the contacts are open, initiating the arc formation process. Under analysis of Fig. 2, the calculation of the MCR is determined by the average value presented in its region. For evaluation of ACR, the area under the AC region (ACA) curve or the mean (ACM) of the values presented by ACR are usually calculated [9]. For a degraded contact, it is expected an alteration on the shape of the resistance curve, since the increase of the degradation can result in mechanical deformations and loss of contact, provoking fluctuations in the measured resistance and the increase of the average resistance values. Therefore, the use of variance as an evaluation parameter can provide complementary information for the diagnosis of the contacts. 3 Methodology 3.1 Material For the development of this work, a medium voltage minimum oil circuit breaker (MOCB), 15 kV voltage class, manufactured by Siemens, model number 3AC2711-4, was used. The circuit break is capable of interrupting currents up to 12.5 kA, and its nominal current of operation is 800 A. Five pairs of contacts (C1, C2, C3, C4 and C5), composed of fixed and mobile contacts, were used in this research. These contact pairs come from MOCB and have different levels of degradation, as presented in Fig. 3. Fig. 3Open in figure viewer Contact samples All the contact samples presented in Fig. 3 were used in the field. However, the samples C3, C4 and C5 were subjected for several short circuits and overloads failures, being considered as highly degraded contacts, while C1 and C2 were not subject to such stressful conditions, presenting low degradation level. Therefore, the C1 and C2 contacts were purchased exclusively for laboratory studies and comparative tests. For voltage and current measurements, a four channel digital oscilloscope from Tektronix, model MDO4104B-6, was used, which has a sampling frequency of 2.5 GS/s and 6 GHz bandwidth. 3.2 Test arrangement Injection of the test current is accomplished by associating a stationary battery (12 V/220 Ah) in series with an adjustable resistor bank (0.040 Ω–1.2 Ω). DRM curves were acquired with an oscilloscope configured to take 100000 samples in 20 milliseconds. The system schematic described is shown in Fig. 4 and presented in a real photo in Fig. 5. Fig. 4Open in figure viewer DRM test arrangement schematic [8] Fig. 5Open in figure viewer DRM laboratory test arrangement The arrangement was adjusted to provide a current of 300 A applied to the terminals of the MOCB pole under test, which was repeated 10 times for each contact. The voltage curve over the opening operation was acquired directly by an oscilloscope channel, while the injected current could be measured with a current transducer, connected in another channel of the oscilloscope. With the current and voltage values obtained, it was possible to calculate the resistance curves, from which the evaluation parameters of the contacts can be extracted. 3.3 DRM parameters calculation For the evaluation of the contacts, resistance curves obtained by DRM tests are usually analysed with the calculation of ACA or ACM. However, other parameters of the DRM curve may influence the estimation of HVCB AC, such as dispersion measurements, or another position measurement, as the median. Since the ACR values vary over time for each DRM curve, each one of the parameters analysed in this paper will be assigned an average calculated among the 10 tests performed. In order to extract the evaluating parameters, an algorithm was developed. The ACR and the MCR were isolated according to the following steps: Calculation of the mean resistance value of the first 1000 points of the dynamic resistance curve (Rm): The Rm value is referred to the MC. Since there is no significant variation of the resistance when the current is flowing through the MCs, 1000 points is enough to represent this value; Identification of the MCs separation instant (t1): After , the resistance values are referred to the ACs. Since there is no significant variation of the resistance when the current is flowing through the MCs, the algorithm identifies when an increase of 5% of Rm is detected; Identification of the ACs separation instant (t2): After there is no more galvanic connection between the fixed and mobile contacts, and it is also the electric arc ignition instant. To define t2, the algorithm needs to detect when the contact resistance tends to infinity. Therefore, the typical values of contact resistance were taken into consideration to establish a threshold parameter for the algorithm. For this purpose, this information can be obtained from the CB manufacturer application guide. According to the manufacturer of the CB used in this research, model 3AC, highly degraded contacts should present a maximum resistance of 600 µΩ in the MCs [21, 22]. Hence, in this case, a value of 2000 µΩ was considered as a good indicator of total galvanic separation. This value was used not only to define t2 in the algorithm, but also to configure the trigger of the oscilloscope; Definition of the ACR: Immediately after there is often an abnormal resistance variation due to mechanical jumps of the mobile contacts. These variations may represent false resistance values and result in a wrong contact diagnosis. Similarly, immediately before , an abnormal resistance variation might be obtained. Therefore, to consider these transition zones, a 10% portion of the t1–t2 interval after t1 and before t2 were not considered. Hence, the ACR interval is defined as: The proposed methodology for the algorithm construction can be expanded to other CBs, since the threshold value can be established accordingly to the typical contact resistance values provided by each CB manufacturer. The following evaluating parameters were calculated considering the ACR interval mentioned above. 3.3.1 Area under AC region (ACA) The ACA calculation is performed by trapezoidal numerical integral of the measured ACR values, according to (1) 3.3.2 Mean of arcing contact resistance (ACM) ACM is calculated as the mean value presented by ACR along its characteristic region, determined by. (2) 3.3.3 Arcing contact resistance median (ACMe) To determine ACMe, we first proceed with the increasing ordering of ACR values. If N is odd, ACMe is represented by the central ACR value. If N is even, ACMe is determined by the mean of the ACM central values. 3.3.4 Amplitude of ACR (ACAm) The ACAm is defined as the difference between the highest and the lowest value of the set of points in the curve. Then, the parameter is calculated as (3) 3.3.5 Arcing contact resistance variance (ACV) The ACV is the dispersion measure defined as the mean square of the deviations of the ACR values along the curve from the mean. Therefore, ACV is calculated as presented below (4) 4 Results Performing a routine elaborated in Matlab® software, according to the algorithm presented in the previous section, the MC and AC regions were delimited for each DRM test results. The same routine calculates the DRM alternative parameters. Since the applied test current value (300 A) is considerably lower than the CB rated current (800 A), the laboratory tests were considered as non-destructive and no significant changes for the DRM curve behaviour of each contact were verified for all the samples. Such as the sample presented in Fig. 2, all the DRM samples presented an abrupt resistance value change for the 18 ms time instant, since the trigger time reference of the oscilloscope was configured for such instant. Therefore, 18 ms was considered as the ACs separation instant t2 defined by the algorithm presented in the previous section. A sample of the DRM curves for each contact evaluated is presented in Fig. 6, in which the abrupt resistance value change (> 5*Rm) around 18 ms can be verified for all the contacts. Fig. 6Open in figure viewer DRM sample curves for the evaluated contacts For the MCs separation instant t1, there were no significant changes between the calculated values for each sample, as presented in Fig. 7. The highest standard deviation (0.2 ms) was verified for the contact C4, while the other contacts presented values <0.1 ms. Therefore, considering t2 = 18 ms, the t1 variations presented in Fig. 7 are not sufficient to significantly impact the t2–t1 interval applied for the ACR definition. Fig. 7Open in figure viewer MCs separation time (t1) for each sample Hence, since no significant changes over the t1 and t2 parameters were verified. The analyses were made considering the mean values for all the conventional evaluation parameters for each contact, as shown in Fig. 8. Fig. 8Open in figure viewer Mean values and error bars (maximum/minimum values) of conventional DRM parameters comparison (a) ACA values, (b) ACM and ACMe values From Fig. 8, it can be seen that the values of ACA do not represent correctly the contacts degradation stage. The contact C2, considered as a contact with low degradation level, present ACA values higher than all the evaluated contacts with high level of degradation (C3, C4 e C5). The same can be stated for the C1 contact (low degradation level), which ACA value is lower only than the value presented by the contact C4. Due to mechanical restrictions, such as: displacement of the contacts; position of the contacts; transition from the MC to the contact of arc and; mechanical defects, the accurate identification of the instant of beginning (t1) of the AC region is a hard task subjected to errors that can influence significantly in the ACA calculation. Small displacements in t1 definition results in high changes in the ACA value. As can be seen in Figs. 6 and 7, the beginning instant of the AC region, is not the same for the evaluated contacts resulting in the incoherence between the ACA values presented in Fig. 8. Similar to the ACA results, the ACM evaluating parameter also do not represent correctly the contacts degradation stage. Again, the contacts C1 and C2 presented higher values than most of the degraded samples. The incoherence between the ACM values presented in Fig. 8 can be attributed to the differences among the ACR values for each contact DRM curve. For C1 and C3, for example, a simple t1 displacement (≃ 1 ms) and the ACR differences between the DRM curves resulted in a significant ACA and ACM errors, respectively, as highlighted in Fig. 9. Fig. 9Open in figure viewer C1 and C3 ACA and ACM representation It can be observed from Fig. 9 that the ACR curve of sample C3 is smaller than that of sample C1 until near the middle of the separation of the contacts. However, due to the high level of degradation in C3, there is a considerable variation of the ACR values in relation to their mean approximately between 12 and 14 ms, resulting in a mean value close to the presented for the C1 contact as highlighted in Fig. 9. The same approach can be used to explain the incoherence presented in the ACMe values too. The abrupt variation presented in the ACR values of the sample C3 is also noticed in the other two degraded contacts (C4 and C5). These variations are possibly consequential effects of cracks and/or prominences existing in the AC, which, therefore, evidence indications of a higher level of degradation. Therefore, the use of tools that can measure these variations can be applied as a good evaluation parameter regarding the AC degradation levels, since the conventional evaluating parameters (ACA, ACM, and ACMe) do not correspond proportionally with the actual degradation state of the analysed contacts. The possible evaluating parameters analysed were the ACAm and ACV along the DRM curve. Fig. 10 presents the ACAm and ACV mean values extracted from the evaluated DRM curves. Fig. 10Open in figure viewer Mean values and error bars (maximum/minimum values) of proposed DRM parameters comparison (a) ACAm values, (b) ACV values From Fig. 10, it can be observed that the ACAm presents a certain correlation between the extracted values and the degradation stage of the contact. The contacts with high degradation level presented higher ACAm values than the contacts with low degradation level, with the exception of C5, that presented lower ACAm value than C2. However, the ACAm value could not be a fully reliable parameter, since the highest values are usually located at the end of the DRM curve, characterised by drastical increases of the resistance, resulting in measurement uncertainties for each DRM curve that can lead to incorrect diagnosis. On the other hand, the variance of the resistance values (ACV) over time may indicate the presence of spurious values resulting from the quality of the insulating medium and/or the AC. All the contacts with high degradation level presented higher ACV values than the contacts with low degradation level, representing a potential evaluating parameter for contacts degradation level diagnosis. Notice that the mentioned conventional evaluating parameters shouldn't be excluded in the analysis about contacts degradation levels. Hence, as presented in this section, conventional parameters could not correspond proportionally with the current degradation state, being pertinent to evaluate different parameters of the DRM curve beyond those usually used and found in the literature. The use of the dispersion parameters (amplitude and variance) along the DRM curve was efficient in estimating the degradation of the samples. Therefore, it is interesting to jointly evaluate more than one parameter associated to DRM. A useful method of analysing more than one attribute at a time is the use of the radar chart, which is shown in Fig. 11. Fig. 11Open in figure viewer DRM parameters radar chart (dashed lines represent the low degraded contacts) The graphic presented in Fig. 11 was achieved through a normalisation process based on the minimum value of each evaluating parameter, followed by an inverse operation in order to display each parameter in a range of 0–1. Therefore, the closer to 0, the higher the contact degradation level. Hence, from the radar chart, it can be observed that the evaluated dispersion parameters (ACAm and ACV) provided a better distinction between the contacts degradation levels. For a practical application, the evaluating parameters obtained for new CB contacts should be used as reference values for the radar chart assembly. Then, during the HVCB operating lifetime, DRM measurements should be made periodically and comparisons between the current evaluating parameters and the reference parameters values would be made in order to verify any change that can be attributed as contact degradation. Therefore, from the radar chart shape and value changes, the contact degradation level can be estimated. However, this tool still depends on the expertise of the maintenance operator of the electric utility, since the DRM method is not established and quantitative values are not defined for CB diagnosis yet. 5 Conclusions DRM is not currently a consolidated technique, so there are no standards or manufacturer-defined parameters for accurate AC classification. However, the DRM is efficient in pointing out the occurrence of continuous degradation, established by comparing different parameters previously measured, between the poles of the same HVCB or between HVCB of the same manufacturing model. From the results obtained in this paper, it can be verified that the use of the area under the DRM curve may not be efficient in the diagnosis of some specific problems, such as the punctual degradation presented by the contacts C3 and C4. Therefore, the application of a continuous and joint evaluation with more than one diagnostic parameter can provide an accurate monitoring of the actual state of AC degradation. In this work, the use of not commonly evaluated parameters, i.e. the variance (ACV) and amplitude (ACAm) of the ACR values, along the DRM curve proved themselves as complementary parameters that can be addressed in the evaluation of the degradation state of the AC, aiding in the DRM technique consolidation process for contacts diagnosis. Among the studied parameters, the ACV was highlighted by presenting the higher correlation with the contact degradation level, being its values in agreement with the visual inspection, in which the contacts C3 and C4 presented the higher degradation levels. Moreover, since the proposed parameters and technique are based only on contact resistance measurement and data processing routine with low complexity and ease of adaptation, its applicability is not restricted to a specific CB type and can be transferred to another CB technologies, such as SF6 and air blast. Lastly, with the inclusion of new and more effective evaluating parameters in the DRM curve analysis a more reliable diagnosis of CB contacts and, consequently, about its lifetime can be achieved. Additionally, through an accurate CB evaluation, maintenance scheduling can be optimised, improving substation asset management. 6 Acknowledgments The authors would like to thank the Post-Graduation Coordination in Electrical Engineering (COPELE), the Coordination for Improvement of Higher Level Personnel (CAPES), the National Council for Scientific and Technological Development (CNPq) and the High Voltage (LAT), from the Federal University of Campina Grande (UFCG). 7 References 1Ryan H., and Jones J.: ' SF6 switchgear' ( Peter Perigrinus Ltd, London, 1989) 2Holm E., Holm R., and Il E.I.S.: 'Theory of hardness and measurements applicable to contact diagnostics', J. Appl. Phys., 1949, 20, (4), pp. 319– 327 3Bagherpoor A., Rahimi S., and Razi-kazemi A.A. et al.: 'Online condition assessment of interruption chamber of gas circuit breakers using arc', Trans. Power Deliv., 2016, 32, pp. 1776– 1783 4Oliveira A.C.: ' Dynamic resistance testing in circuit breakers: analysis of current sensitivity and contact opening speed', Master Thesis, UFCG, Campina Grande, 2015 5Landry M., Turcotte O., and Brikci F.: 'A complete strategy for conducting dynamic contact resistance measurements on HV circuit breakers', IEEE Trans. Power Deliv., 2008, 23, (2), pp. 710– 716 6Ohlen M., Dueck B., and Wwenli H.: ' Dynamic resistance measurements – a tool for circuit breaker diagnostics'. Stockholm Power Tech Int. Symp. on Electric Power Engineering, Stockholm, Sweden, 1995, pp. 108– 113 7Sodha N., Singh S., and Victor S. et al.: ' Condition assessment of EHV class circuit breakers using dynamic contact resistance measurement technique'. Proc. Cigre Session A3–205, Paris, France, 2012, p. 11 8Souza R.T.: ' Contact circuit breaker diagnostics based on dynamic contact resistance and fuzzy logic', Doctorade Tesis, UFCG, Campina Grande, 2015 9 ANSI/IEEE STD C37.09.: ' Test procedure for ac high-voltage circuit breakers', 1999 10 IS/IEC 62271–1.: ' High-voltage switchgear and controlgear, part 1: commom specifications', 2007 11Claesson L., Middleton A., and Guha C.: ' Improving efficiency and safety in HV circuit breaker: how should India embrace best developed practice?', GridTech, 2007 12Landry M., Mercier A., and Ouellet G. et al.: ' A new measurement method of the dynamic contact resistance of HV circuit breakers'. 2005/2006 IEEE PES Transmission and Distribution Conf. and Exhibition, Dallas, USA, 2006, pp. 1002– 1009 13Secic A., and Milovic B.: ' Dynamic resistance measurement method applying high DC current'. Circuit Breaker Test & Maintenance WS (CEATI), Palm Desert, USA, 2014 14Souza R.T., Costa E.G., and Oliveira A.C. et al.: ' Characterization of contacts degradation in circuit breakers through the dynamic contact resistance'. Proc. IEEE PES Transmiss. Distrib. Conf. Expo., Medellin, Colombia, 2014 15Pancu C., Baraboi A., and Adam M.: 'New approach regarding the electroerosion estimation of the circuit breakers contacts', WSEAS Trans. Circuits Syst., 2009, 8, pp. 157– 166 16Jeyaraj S.G., and Habtay Y.: ' Effective and efficient circuit breaker analysis'. IET Conference on Reliability of Transmission and Distribution Networks (RTDN 2011), London, UK, 2011 17Chen G., Lan L., and Pan Z. et al.: 'Electrical erosion test and condition assessment of SF6 CB contact sets', IET Gener. Transm. Distrib., 2017, 11, (8), pp. 1901– 1909 18Liu Y., Zhang G., and Yang J. et al.: 'The mathematical model of contact resistance and injected current in DRM tests of SF6 circuit breaker', IEEE Trans. Compon. Packag. Manuf. Technol., 2018, 8, (1), pp. 82– 87 19Khoddam M., Sadeh J., and Pourmohamadiyan P.: 'Performance of circuit breaker electrical contact based on dynamic resistance signature and using health Index', IEEE Trans. Compon. Packag. Manuf. Technol., 2018, 6, (10), pp. 1505– 1512 20Abdollah M., and Kazemi A.A.R.: 'Intelligent failure diagnosis for gas circuit breakers based on dynamic resistance measurements', IEEE Trans. Instrum. Meas., 2018, 68, pp. 3066– 3077 21 SIEMENS.: ' 3AC circuit breaker (15 kV) application guide', Siemens Power Transmission and Distribution Inc., Richland, 1986 22Souza R.T.: ' Diagnóstico de Contatos de Disjuntores Baseado na Resistência Dinâmica de Contato e Lógica Fuzzy', Doctoral dissertation, Brazil, 2015 Citing Literature Volume4, Issue3September 2019Pages 197-202 FiguresReferencesRelatedInformation
Referência(s)